The Physics World 2023 Breakthrough of the Year goes to a Swiss–French team led by Grégoire Courtine at Ecole Polytechnique Fédérale de Lausanne (EPFL), Jocelyne Bloch at Lausanne University Hospital and EPFL and Guillaume Charvet at CEA-Leti for the development of a digital bridge that restores communication between the brain and spinal cord. When tested on a man with paralysis, the system enabled him to stand and walk naturally.
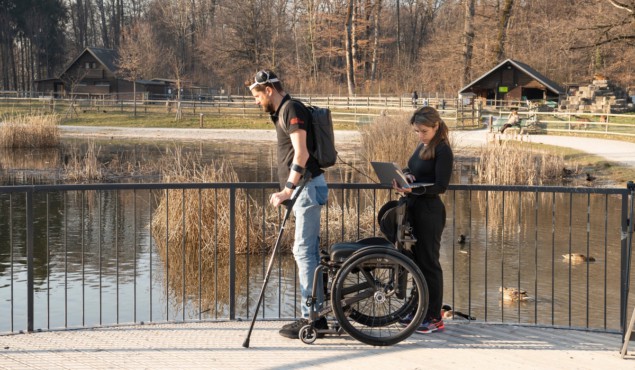
Spinal cord injury can disconnect communication between the brain and the region of the spinal cord that produces walking, which can lead to permanent paralysis. To restore this communication, the team developed a brain–spine interface, comprising two implantable systems: one to record cortical activity and the other to electrically stimulate the region of the spinal cord that controls leg movement.
To monitor signals from the brain, a 64-channel grid of electrodes is implanted in the participant’s brain in regions that respond to the intention to move the lower limbs. An artificial intelligence-based algorithm then decodes these brain signals in real time, to predict the user’s motor intentions, and converts them into stimulation commands to activate leg muscles.
The second device is a neurostimulator connected to an electrode array, which is implanted over the region of the spinal cord that controls leg movement. This device delivers the required electrical stimulation to activate leg muscles – essentially creating a digital bridge between the brain and spinal cord. The whole system operates wirelessly, allowing a user to move around independently.
The team tested the system in a 38-year-old male with a spinal cord injury from a bike accident 10 years earlier. Following implant surgery, the bridge enabled the participant to regain intuitive control over his leg movements, enabling him to stand, walk with crutches, climb stairs and traverse complex terrains. The brain–spine interface remained reliable and stable for over one year of use, including at home without supervision.
This research brings hope to people with spinal-cord injuries and that is why we are very pleased to call it our Breakthrough of the Year for 2023.
Selection criteria
The Physics World 2023 Breakthrough of the Year was selected by a panel of Physics World editors, who sifted through hundreds of research updates and news stories published on the website this year across all fields of physics. In addition to having been reported in Physics World in 2023, the winner must meet the following criteria:
- Significant advance in knowledge or understanding
- Importance of work for scientific progress and/or development of real-world applications
- Of general interest to Physics World readers
The nine runners-up that complete our Top 10 Breakthroughs for 2023 are listed below in chronological order of when they were reported in Physics World.
Growing electrodes inside living tissue
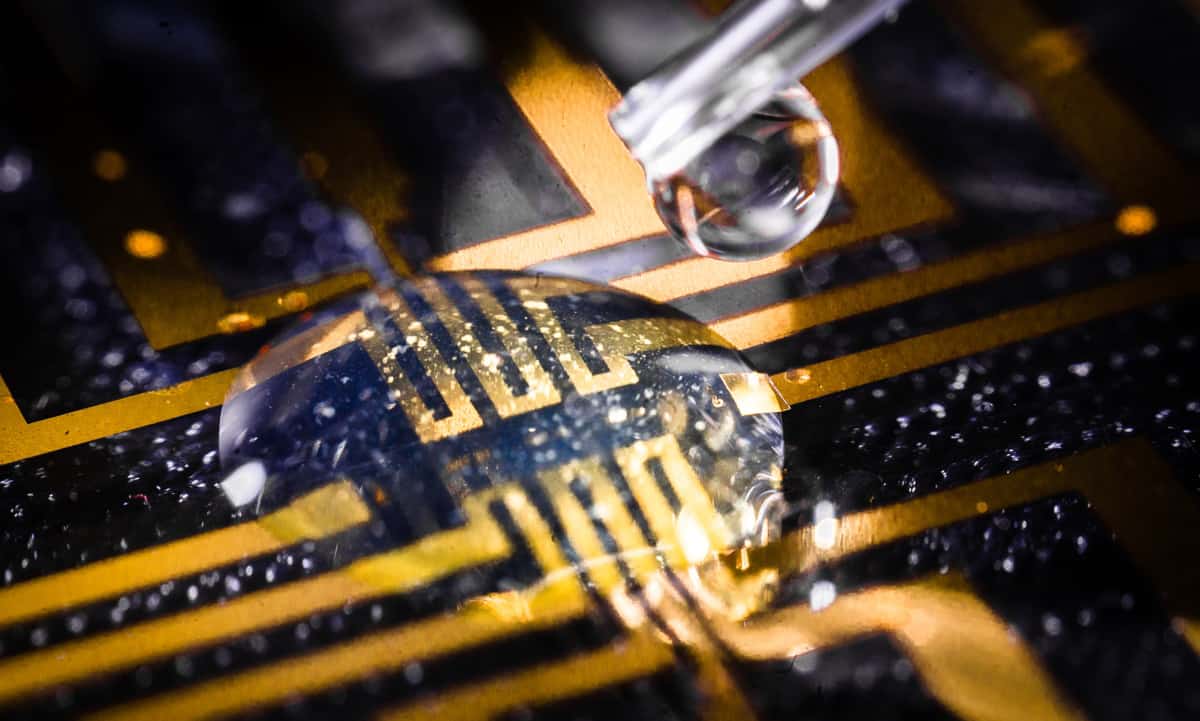
To Xenofon Strakosas, Hanne Biesmans, Magnus Berggren and colleagues at Linköping University, Lund University and the University of Gothenburg for developing a way to create electronic circuits directly inside living tissue. Interfacing neural tissue with electronics provides a way to study the complex electrical signalling of the nervous system or modulate neural circuitry to treat disease. However, the mismatch between rigid electronics and soft tissues risks damaging delicate living systems. Instead, the team used an injectable gel to create soft electrodes directly within the body. After injection into living tissue, enzymes in the gel break down endogenous metabolites in the body, which trigger enzymatic polymerization of organic monomers in the gel, converting them into stable, soft conducting electrodes. The researchers validated the process by injecting gels into zebrafish and medicinal leeches, where the gel polymerized and grew electrodes within the tissue.
Neutrinos probe the proton’s structure
To Tejin Cai at the University of Rochester in the US and Canada’s York University, and colleagues working on Fermilab’s MINERvA experiment for showing how information about the internal structure of the proton can be gleaned from neutrinos scattering from a plastic target. Neutrinos are subatomic particles that are famous for rarely interacting with matter. So, there were doubts when Cai, a postdoctoral researcher, suggested that the occasional scattering of neutrinos from protons in plastic could be observed. The big challenge for the team was observing the signal from neutrinos scattered from lone protons (hydrogen nuclei) within the much larger background of neutrinos scattered off protons bound-up in carbon nuclei. To solve this problem, they simulated the carbon-scattered signal and carefully subtracted it from the experimental data. As well as providing insights into the structure of the proton, the technique could also shed further light on how neutrinos interact with matter.
Simulating an expanding universe in a BEC
To Celia Viermann and Markus Oberthaler of the University of Heidelberg, Germany, together with Stefan Floerchinger of the University of Jena, Germany, and colleagues at the Universidad Complutense de Madrid, Spain, Ruhr-Universität Bochum, Germany and the Université libre de Bruxelles, Belgium, for using a Bose–Einstein condensate (BEC) to simulate an expanding universe and the quantum fields within it. In this simulated system, the condensate represented the universe, while phonons moving through it played the role of the quantum fields. By changing the scattering length of the atoms in the BEC, the team made the “universe” expand at different rates and studied how the phonons seeded density fluctuations within it. Theories of cosmology predict that similar effects were responsible for seeding large-scale structure in the early universe, so the simulated universe may produce valuable insights into how the real one came to be the way it is today.
A double slit in time
To Romain Tirole and Riccardo Sapienza at Imperial College London and colleagues for the demonstration of Young’s double-slit interference in time. The 19th-century observation of the interference of light waves by Thomas Young is one of the most iconic experiments in the history of physics and provided fundamental support to the wave theory of light. While that experiment and others like it involve diffraction of light through a pair of narrow slits in space, researchers in the UK and elsewhere showed it is possible to achieve the equivalent effect using double slits in time. The temporal analogue involves fixed momentum but changing frequency. A material in which two slits rapidly appear and then disappear, one after the other, should cause incoming waves to maintain their path in space but spread out in frequency. The researchers achieved this by turning the reflectivity of a semiconductor mirror on and off twice in quick succession and recording interference fringes along the frequency spectrum of light bounced off the mirror. They saw that the interference happens between waves at different frequencies – rather than different spatial positions. The work could have several applications such as optical switches for signal processing and communication or in optical computing.
Building blocks for a large-scale quantum network

To Ben Lanyon and colleagues at the University of Innsbruck, Austria, and the University of Paris-Saclay, France, for constructing a quantum repeater and using it to transfer quantum information over a distance of 50 km via standard telecommunications fibres, thereby demonstrating all the key functionalities of a long-distance quantum network in a single system. The team created its quantum repeater from a pair of trapped calcium-40 ions that emit photons after being illuminated with a laser pulse. These photons, each of which is entangled with its “parent” ion, are then converted to telecoms wavelengths and sent down separate 25-km-long optical fibres. Finally, the repeater swaps the entanglement on the two ions, leaving two entangled photons 50 km apart – roughly the distance required to create large-scale networks with multiple nodes.
First X-ray image of a single atom
Saw Wai Hla, Volker Rose at Argonne National Laboratory in the US and colleagues for imaging a single atom with synchrotron X-rays. Until recently, the smallest sample size that could be analysed using synchrotron X-ray scanning tunnelling microscopy was an attogram, which is around 10,000 atoms. This is because the X-ray signal produced by a single atom is extremely weak and conventional detectors are not sensitive enough to detect it. To get around this, the team added a sharp metallic tip to a conventional X-ray detector, which is placed just 1 nm above the sample to be studied. As the sharp tip is moved across the surface of a sample, electrons tunnel through the space between the tip and the sample, creating a current and this essentially detects “fingerprints” that are unique to each element. This allowed the team to combine the ultrahigh-spatial resolution of scanning tunnelling microscopy with the chemical sensitivity provided by intense X-ray illumination. The technique could lead to applications in material design as well as in environmental science through the ability to trace toxic materials down to extremely low levels.
“Smoking gun” evidence of early galaxies transforming the universe
To the EIGER Collaboration for using the James Webb Space Telescope (JWST) to find compelling evidence that early galaxies were responsible for the reionization of the early universe. Reionization occurred about 1 billion years after the Big Bang and involved the ionization of hydrogen gas. This allowed light that would have been absorbed by hydrogen to travel to the telescopes of today. Reionization appears to have begun as local bubbles that grew and coalesced. These bubbles would have been created by sources of radiation, and one possibility is that it came from stars in galaxies. The EIGER researchers used the JWST’s Near Infrared Camera to look at light from ancient quasars that had passed through the ionized bubbles. They found a correlation between the locations of galaxies and the bubbles, suggesting that light from these early galaxies was indeed responsible for reionization.
Supersonic cracks in materials
To Meng Wang, Songlin Shi and Jay Fineberg of the Hebrew University of Jerusalem, Israel, for discovering that cracks in certain materials can spread faster than the speed of sound. The result contradicts both previous experimental results and predictions based on classical theory, which state that supersonic crack propagation should not be possible because the speed of sound in a material reflects how quickly mechanical energy can move through it. The team’s observations may indicate the presence of so-called “supershear” dynamics governed by different principles than those that guide classical cracks, as predicted by Michael Marder of the University of Texas at Austin, US nearly 20 years earlier.
Antimatter does not fall up

To the ALPHA Collaboration for showing that antimatter responds to gravity in much the same way as matter. The physicists used the ALPHA-g experiment at CERN to make the first direct observation of free-falling antimatter atoms – antihydrogen that comprises an antiproton bound to an antielectron. This was done in a tall cylindrical vacuum chamber in which antihydrogen was first held in a magnetic trap. The antihydrogen was released from the trap and allowed to annihilate at the walls of the chamber. The team found that more annihilations occurred below the release point than above it. After considering the thermal motion of the antihydrogen, the team concluded that antimatter falls down. Tantalizingly, the antihydrogen’s acceleration due to gravity was about 75% of that experienced by normal matter. Although this measurement has a low statistical significance, it leaves the door open to new physics beyond the Standard Model.
Honourable mention
Fusion energy breakthrough
An honourable mention in our top 10 for this year goes to physicists working at the $3.5bn National Ignition Facility (NIF) in the US for work that was performed at the lab late last year after we picked our 2022 winners (and so misses out on our 2023 breakthrough choice too). On 13 December 2022 the lab announced the generation of more energy from a controlled nuclear fusion reaction than was needed to power the reaction. The laser shot, performed on 5 December 2022, released 3.15 million joules (MJ) of energy from a tiny pellet containing two hydrogen isotopes – compared to the 2.05 MJ that those lasers delivered to the target. This demonstration of net energy gain marks a major milestone in laser fusion.
- You can listen to a lively discussion about the Top Ten Breakthroughs in this episode of the Physics World Weekly podcast.
Physics World‘s coverage of the Breakthrough of the Year is supported by Reports on Progress in Physics, which offers unparalleled visibility for your ground-breaking research.