
If you want to make a clock, all you need is an oscillation – preferably one that is stable in frequency and precisely determined. Many systems will fit the bill, from the Earth’s rotation to pendulums and crystal oscillators. But if you want the world’s most precise clock, you’ll need to go to the US state of Colorado, where researchers from JILA and the University of Colorado, Boulder have measured the frequency of an optical lattice clock (OLC) with a record-low systematic uncertainty of 8.1 × 10−19 – equivalent to a fraction of a second throughout the age of the universe.
OLCs are atomic clocks that mark the passage of time using an electron that oscillates between two energy levels (the ground state 1S0 and clock state 3P0) in an atom such as strontium. The high frequency and narrow linewidth of this atomic transition makes these clocks orders of magnitude more precise than the atomic clocks used to redefine the second in 1968, which were based on a microwave transition in caesium atoms.
The high precision of OLCs gives them the potential to unlock technologies that can be used to sense quantities such as distances, the Earth’s gravitational field and even atomic properties such as the fine structure constant at extremely small scales. To achieve this precision, however, they must be isolated from external effects that can cause them to “tick” irregularly. This is why the atoms in an OLC are trapped in a lattice formed by laser beams and confined within a vacuum chamber.
An OLC that is isolated entirely from its environment would oscillate at the constant, natural frequency of the atomic transition, with an uncertainty of 0 Hz/Hz. In other words, its frequency would not change. However, in the real world, temperature, magnetic and electric fields, and even the collisional motion of the atoms in the lattice all influence the clock’s oscillations. These parameters therefore need to be very well controlled for the clock to operate at maximum precision.
Controlling blackbody radiation
According to Alexander Aeppli, a PhD student at JILA who was involved in setting the new record, the most detrimental environmental effect on their OLC is blackbody radiation (BBR). All thermal objects – light bulbs, human bodies, the vacuum chamber the atoms are trapped in – emit such radiation, and the electric field of this radiation couples to the atom’s energy levels. This causes a systematic shift that translates to an uncertainty in the clock’s frequency.
To minimize the effects of BBR, Aeppli and colleagues enclosed their entire system, including the vacuum chamber and optics for creating the clock, within a temperature-controlled box equipped with numerous temperature sensors. By running temperature-stabilized liquid around different parts of their experimental apparatus, they stabilized the air temperature and controlled the vacuum system temperature.
This didn’t completely solve the problem, though. BBR shift is the sum of a static component that scales with the fourth power of temperature and a dynamic component that scales with higher powers. Even after limiting the lab’s temperature fluctuations to a few millikelvin per day, the team still needed to carry out a systematic evaluation of the shift due to the dynamic component.
For this, the JILA-Boulder researchers turned to a 2013 study in which physicists in the US and Russia found a correlation between the uncertainty of the BBR shift and the lifetime of an electron occupying a higher-energy state (3D1) in strontium atoms. By measuring the lifetime of this 3D1 state, the team was able to calculate an uncertainty of 7.3 × 10−19 in the BBR shift.
To fully understand the atoms’ response to BBR, Aeppli explains that they also needed to measure the strength of transitions from the clock states. “The dominant transition that is perturbed by BBR radiation is at a relatively long wavelength,” he says. “This wavelength is longer than the spacing between the atoms, meaning that atoms can behave collectively, modifying the physics of this interaction. It took us quite some time to characterize this effect and involved almost a year of measurements to reduce its uncertainty.”
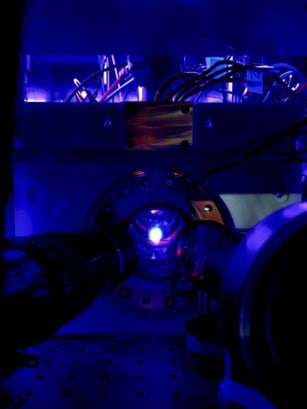
Other environmental effects
BBR wasn’t the only environmental effect that needed systematic study. The in-vacuum mirrors used to create the lattice tend to accumulate electric charges, and the resulting stray electric fields produce a systematic DC Stark shift that changes the clock transition frequency. By shielding the mirrors with a copper structure, the researchers reduced these DC Stark shifts to below the 1 × 10−19 uncertainty level.
OLCs are also sensitive to magnetic fields. This is due to the Zeeman effect, which shifts the energy levels of an atom by different amounts in the presence of such fields. The researchers chose the least magnetically sensitive sub-states to operate their clock, but that still leaves a weaker second-order Zeeman shift for them to calibrate. In the latest work, they reached an uncertainty in this second-order Zeeman shift of 0.1 × 10−18, which is a factor of two smaller than previous measurements.
Even the lattice beams themselves cause an unwanted shift in the atoms’ transition frequency. This is known as the light or AC Stark shift, and it is due to the power of the laser beam. The researchers minimized this shift by ramping down the beam power just before starting the clock, but even at such low trapping powers, atoms in the different lattice sites can still interact, and atoms at the same site can collide. These events lead to a tunnelling and a density shift, respectively. While both are rather weak, the team nevertheless investigated their effect on the clock’s uncertainty and constrained them to below the 10−19 level.
How low can you go?
In early 2013, JILA scientists reported a then-record-low systematic uncertainty in their strontium OLC of 6.4 × 10−18. A year later, they managed to reduce this uncertainty by a factor of three, to 2.1 × 10−18. Ten years on, however, progress seems to have slowed: the latest uncertainty record improves on this value by a mere factor of two. Is there an intrinsic lower bound?
“The largest source of systematic uncertainty continues to be the BBR shift since it goes as temperature to the fourth power,” Aeppli says. “Even a small reduction in temperature can significantly reduce the shift uncertainty.”
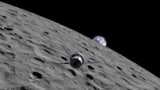
Atomic clocks on the Moon could create ‘lunar positioning system’
To go below the 1 × 10−19 level, he explains that it would be advantageous to cool the system to cryogenic temperatures. Indeed, many OLC research groups are using this approach for their next-generation systems. Ultimately, though, while progress on optical clocks might not be quite as fast as it was 20 years ago, Aeppli says there is no obvious “floor”, no fundamental limit to the systematic uncertainty of optical lattice clocks. “There are plenty of clever people working on pushing uncertainty as low as possible,” he says.
The JILA-Boulder team reports its work in Physical Review Letters.