As Mars nears its closest-ever position to Earth, a series of forthcoming space missions is set to revolutionize our understanding of the surface and atmosphere of the red planet. The missions could even solve the mystery of whether life once existed on Mars
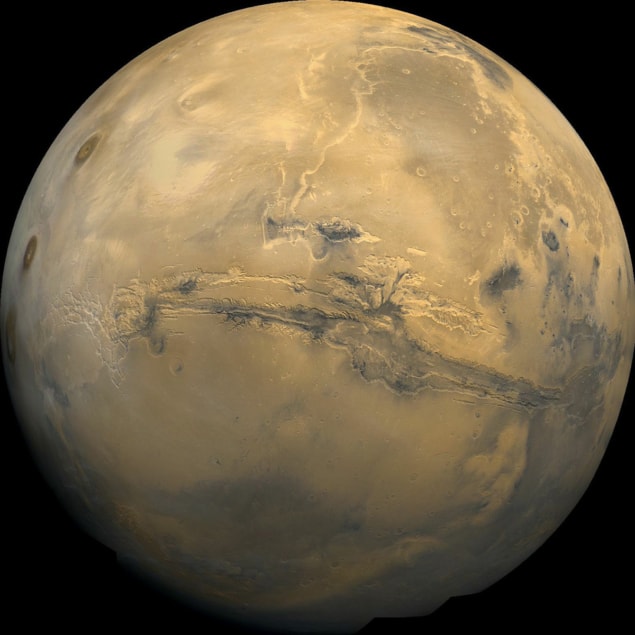
One of the most profound discoveries that has emerged from the past 40 years of space exploration is that environmental conditions like those that we enjoy on Earth are exceedingly rare. However, there is a short list of special places in our solar system that astronomers believe may once have been — or may still even be — very much Earth-like. Mars is at the top of that list.
Today Mars is a cold, bone-dry and inhospitable world with a windswept and sterile surface. However, telescopic data, space missions, and studies of meteorites that are thought to have come from Mars paint a very different picture of the first few billion years of the planet’s history. Compelling evidence exists that the atmospheric pressure and temperature on early Mars may have — at least occasionally been much higher than at present. They could even have been high enough for liquid water to have been stable for long periods on the surface and to have played an important role in the planet’s geologic and climatic evolution.
But much of the evidence is ambiguous, and many crucial questions remain. Was early Mars really “warm and wet” as some astronomers have hypothesized? Did environmental conditions change slowly, rapidly or even episodically over time? What happened to the water that once flowed in substantial volumes across the surface? Perhaps most importantly, was early Mars a habitable world, and — if so — did life ever form, exist or evolve there?
Exploring Mars and attempting to answer these questions has not been easy. Since the Soviet Union launched the Marsnik mission in 1960, the space-faring nations of the world have sent more than 35 missions to fly-by, orbit, land or rove on the red planet. Fewer than half of these, however, have been successful, due to a combination of mechanical and human errors or just plain bad luck. Despite the frequent setbacks, we have persevered and the probes that have succeeded have returned a wealth of data on the planet’s surface, atmosphere and interior conditions. These data have yielded a string of discoveries and surprises that have motivated and inspired researchers to continue exploring our enigmatic planetary neighbour (figure 1).
Early discoveries
Centuries of telescopic observations revealed Mars to have a dynamic surface and atmosphere. Spectroscopic observations that were carried out in the 1940s and 1950s allowed the composition, pressure and temperature of the Martian atmosphere to be determined for the first time. We now know that it consists mainly of carbon dioxide at an average temperature of 250 K (or 23 oC) and a surface pressure of 510 millibar. Imaging and spectroscopic observations at visible to near-infrared wavelengths in the 1950s and 1960s also provided basic information about the surface of the planet.
Bright, reddish areas appeared to consist of highly oxidized ferric (Fe3+) minerals. Darker, reddish regions seemed to be made up of less-oxidized ferrous (Fe2+) volcanic minerals, while the bright, seasonal polar caps appeared to contain frozen water and, possibly, solid carbon dioxide. Although these discoveries pushed telescope technology to its limits, the observations were fundamentally limited by the relatively low spatial resolution that could be achieved with ground-based observations. At best, the smallest details that could reliably be studied on Mars were still many hundreds of kilometres across.
Up-close spacecraft exploration of Mars began with NASA’s Mariner 4, 6 and 7 fly-bys in the 1960s. These missions were spectacular technical achievements, but they provided only a glimpse of the planet’s complexity and mystery. Perhaps the most significant discovery based on data returned from these missions was made in 1966 by the physicist Robert Leighton and the planetary geologist Bruce Murray, both of whom were at the California Institute of Technology. They realized that the temperature and pressure at the Martian surface were such that the carbon dioxide in the atmosphere was in equilibrium with that on the surface. This prediction and others have been confirmed by subsequent missions. They have shown that Mars today has a unique climate that is dominated by the condensation and sublimation of its major atmospheric constituent, carbon dioxide.
The first spacecraft to orbit Mars — Mariner 9 in 1971 and the twin Viking orbiters in 1976 — confirmed the existence of solid carbon dioxide in the polar caps. These missions also showed that Mars is not just a mere reddish point of light in the sky, but a real place with interesting geology and a dynamic atmosphere. Indeed, Mariner and Viking revealed Mars to be a planet of solar-system superlatives: it has the highest mountains, the longest canyons and the largest dust-storms. The southern hemisphere appeared to be ancient, dominated in many places by a rugged Moon-like landscape of tightly packed impact craters. The northern hemisphere, meanwhile, had relatively few craters and therefore appeared much younger. It is also smoother, flatter and dominated in places by enormous volcanoes with extensive lava-flow systems.
Other measurements from the Viking orbiters confirmed that Mars’ current atmosphere is very dry. If all the water vapour in the Martian atmosphere were condensed and spread out as a layer of water across the planet, it would form a film just 10 µm thick. (Doing the same to the Earth’s water, in contrast, would create a layer tens of metres deep.) Despite the planet’s current dryness, the Viking images revealed stunning evidence for several kinds of apparently water-carved features that could only have formed if the Martian atmosphere had at some point been warmer and at higher pressure. Indeed, on closer inspection many of the heavily cratered regions showed evidence of erosion patterns that also seemed consistent with thicker atmospheric conditions in the past. Some astronomers even suggested that various features in the images might be the shoreline of an ancient ocean that once spanned almost the entire northern hemisphere (figure 2).
While the Viking orbiters were revealing mysteries from high above, two landers sent to the surface by the spacecraft set about chronicling the surface conditions in detail for the first time. The Viking landers set down on Mars in 1976 and imaged dusty, rock-strewn landscapes bathed in the reddish cast of a highly scattering, dusty atmosphere. Pressure sensors on the landers operated for several Martian years and recorded seasonal variations of more than 25% in the surface pressure — twice that seen during the strongest hurricanes on Earth. These results confirmed that substantial exchange of surface and atmospheric carbon dioxide does indeed occur semi-annually in the polar regions. (One Martian year lasts 687 Earth days.)
However, the main scientific focus of the landers was not geology or meteorology but biology. A number of experiments were conducted to search for evidence of organic molecules in the soil and dust. Could any organisms or complex organic molecules convert nutrients that had been brought from Earth into usable biological energy? Were primitive forms of “respiration” occurring on the surface? By most measures, the experiments that searched for life or complex organic chemistry on Mars were all negative. Non-organic surface chemistry was instead invoked to explain most of the results obtained. This lack of organic molecules at the part-per-billion level in the soil and dust has since been found to be consistent with the lack of an ozone layer on Mars. This is perhaps not surprising, as the surface is constantly exposed to intense solar ultraviolet radiation, which would break down the carbonhydrogen bonds in any organic molecules.
A rock and a rover
Results from the Viking landers seemed to dash the hopes of those who thought of Mars as a possible abode for extraterrestrial life. But attitudes swung back the other way in 1996. That year a team of NASA scientists stunned the world by unveiling possible evidence for fossilized life forms preserved in a meteorite that was believed to have come from Mars. The rock in question, known as ALH84001, is one of 20 or so currently known meteorites hypothesized to have made their way to Earth, after having been blasted off Mars by relatively recent asteroid or comet impacts. The Martian origin of these rocks is based on measured or inferred isotopic and geochemical similarities to soil and rocks from Mars that have been studied in situ.
ALH84001 is the oldest known Mars meteorite by far, having been created just a few billion years after the planet was formed. The rock was meticulously examined by a team of researchers led by David McKay, a planetary geochemist at NASA’s Johnson Space Center in Houston, Texas, who studied its geochemical, magnetic, organic and morphologic properties. McKay and colleagues became convinced that the rock preserves evidence of ancient bacteria-like organisms that once thrived on the red planet.
Counter-arguments against a biological origin for each of these pieces of evidence have subsequently been published, and meteorite scientists remain bitterly divided over the issue. Rather than take sides on a debate that may be impossible to resolve given the available data, many astronomers in-stead focus on a less-ambiguous observation. We know that ALH84001 contains indigenous complex organic molecules such as polycyclic aromatic hydrocarbons chains of carbon and hydrogen atoms like those that exist in many dense interstellar molecular clouds. The rock’s geochemistry and mineralogy also strongly suggest to astronomers that it was heated substantially early on in its existence and that liquid water has coursed through its veins. These three factors –the presence of organic molecules, a heat/energy source, and liquid water — are exactly what is needed if a planet is to be hospitable for the formation or evolution of life as we know it.
While the debate over ALH84001 was raging, NASA was getting back into the business of probing the surface of Mars. In summer 1997 it sent the Mars Pathfinder lander and rover to the mouth of the Ares Valles outflow channel. The landing site — about 20° north and 35° west — was selected after the Viking mission found evidence that a variety of rock types might have been transported to this region by large floods early in the planet’s history. But Pathfinder was not really a scientific project. It was primarily a technology-demonstration mission, designed to validate NASA’s new “better, faster, cheaper” approach to planetary-exploration missions. It was also meant to identify technologies that could be used in future landed missions. Nevertheless, Pathfinder was phenomenally successful. It also tapped directly into the general public’s interest in space exploration by streaming out images and other data to the world in near-real-time using a then relatively new medium called the World Wide Web (figure 3).
In addition to the engineering and public-relations successes, Pathfinder also produced a number of important and new scientific results that enhanced our understanding of Mars. The mission included a small, semi-autonomous rover called Sojourner, which took close-up images and measured the elemental chemistry of soils and rocks. The relative proportions of the elements that it determined were similar to those obtained from soil and dust by the Viking missions back in the 1970s. This confirmed that in many places Mars is covered with fine-grained materials, including at least one common component found throughout the planet’s surface.
However, the soil samples collected by Pathfinder and Viking were found to contain very different amounts of sulphur and chlorine. Another unusual discovery made by the Sojourner rover was the big variation in the amount of silicon contained in different rocks. These results have not yet been fully explained, but they may reflect differences in the chemical weathering of rocks and soil at the Pathfinder site compared with elsewhere on the planet. Another possibility is that the source region for what are thought to be primarily volcanic rocks at the site had a substantially different chemistry and/or eruptive history compared with other areas of the planet.
One of the most intriguing results from the Pathfinder mission was obtained by dynamicist William Folkner and colleagues from the Jet Propulsion Laboratory at Caltech. They carefully monitored the Doppler shift of the lander’s radio signal as it was received on Earth and then deconvolved from that a highly accurate measure of the current rotation period of Mars. By comparing the period to similar measurements made over 20 years earlier by the Viking landers, they were able to determine the planet’s precession rate and thus its moment of inertia. Armed with a value for the moment, the researchers could then estimate the size of the Martian core and mantle. Although the results depend on the specific composition and density (in particular the ratio of iron to sulphur) of the core, the data are consistent with the planet having a solid and relatively large core with a radius that is 40-60% of the total radius.
New missions, new puzzles
After recovering from the unfortunate loss of the Mars Observer spacecraft due to mechanical failure just before its arrival at the planet in 1993, NASA got back into the Mars orbiter business in 1996. In November of that year, it successfully launched the Mars Global Surveyor (MGS) spacecraft, just weeks before Pathfinder blasted off. MGS, which carries wide-angle and high-resolution cameras, an infrared spectrometer and a laser altimeter, as well as a sensitive magnetometer, has been making orbital remote-sensing measurements of the planet since 1997 — a period of more than two Martian years.
MGS has completely revolutionized our view of Mars. The planet’s topography has been mapped globally and is now known better than that of the Earth! The topography, along with gravity-field data derived from analysis of the spacecraft’s orbital motion, reveals that the crust of the southern highlands is about twice as thick as that of the northern lowlands. The silicate mineralogy of the surface has been mapped globally by the MGS infrared spectrometer, providing details of the composition and compositional variations of volcanic rocks on the planet. Interestingly, however, no evidence for carbonate rocks has yet been found, even though these minerals were once thought to be the reservoir where carbon dioxide from a thicker, ancient atmosphere could have been stored.
MGS’s spectrometer has also been used to monitor thermal emission from the atmosphere, providing daily maps of the temperature of the Martian atmosphere and plots of the levels of dust and water. In the summer of 2001 it made an unprecedented series of measurements during what was the largest planet-encircling dust storm seen on Mars in more than 30 years. The results from these infrared observations have been enhanced and extended by near-simultaneous ultraviolet and visible measurements of Mars that several colleagues and I have been making with the Hubble Space Telescope. Hubble provides a global weather-satellite-like view of the planet that cannot be obtained from low-orbiting spacecraft like MGS. Hubble’s short-wavelength images and spectra complement the data from MGS on the photochemistry of the atmosphere, diurnal variations in aerosols, and composition of highly altered surface minerals (figure 4).
While global measurements of the planet’s magnetic field show that Mars does not have an internally generated dipole field at present, fairly intense magnetic anomalies — both isolated and in broad zones of alternating polarity — have been detected on the surface by MGS. These anomalies appear to be evidence for preserved magnetism in crustal rocks from a time in the distant past when Mars had an internal field and an active core dynamo. Additional, spectacular evidence supporting a more active and dynamic geological past for Mars comes from the high-resolution images being obtained by MGS. These images, which have a resolution that is over ten times better than those from the Viking orbiter, reveal a complex and unexpected variety of both active and ancient landforms and processes.
Arguably one of the two most exciting discoveries from the MGS images is that the uppermost few kilometres of the Martian surface have a layered structure throughout the planet. This indicates that the upper part of the crust is not just a jumbled-up melange of impact-generated fragments, as on the Moon, but is instead a stack of apparently sedimentary deposits that were laid down during substantially different environmental and depositional conditions. It is not known if the deposits were formed from volcanic eruptions or whether they were created by wind or water. However, there is evidence that all three of these processes are good candidates in different places. Many of the layers have been heavily eroded through time, and some regions appear to have had many episodes of erosion and deposition in which the ancient underlying bedrock deposits were alternately exposed, buried and re-exposed in turn.
The other exciting discovery to have been made with MGS was reported in 2000 by the planetary geomorphologists Michael Malin and Kenneth Edgett of Malin Space Science Systems Inc. They found striking evidence that liquid water once flowed across the surface of Mars in the geologically recent past perhaps in the last few million years or less — and that it may still exist even today in some places just under the surface. The water appears to have emanated from the walls of impact craters and other steep-sided landforms, manifesting itself as small, extremely fresh-looking gullies and channels. This result seems counterintuitive, as the current pressure and temperature of the atmosphere are outside the liquid part of the phase curve of water.
Models by Malin and Edgett — as well as others like those by planetary scientists Michael Mellon of the University of Colorado and Roger Phillips of Washington University — propose that the water emerged after being held under pressure in the sub-surface. Even given current surface conditions, the water could flow for hundreds of metres or more, depending on the volume and rate of flow, before completely subliming into the atmosphere. This interpretation is, however, not conclusive, and alternative explanations have been proposed for the small gullies, including wind erosion, landslides and the release of liquid carbon dioxide. The simplest explanation appears to be that shallow layers of liquid water once existed on Mars — at least in a few places on the planet. This explanation also has the most interesting and exciting implications for the future of Mars exploration (figure 5).
More recent support for the existence of shallow sub-surface water on Mars has come from measurements by the latest spacecraft to reach the planet — the NASA Mars Odyssey orbiter. Odyssey was launched in 2001, laden with visible and infrared cameras, neutron spectrometers and high-energy gamma-ray detectors, all of which are designed to determine and map the composition of the planet’s surface. Odyssey’s mapping mission began in early 2002, and the imaging systems have been returning spectacular data at resolutions of 20100 m. This lies between the resolution of hundreds of metres obtained with the Viking mission and the 1.55 m of the MGS camera.
The most exciting result to date, however, has come from the high-energy experiments. These involve measuring the secondary neutrons and gamma rays that are generated when galactic cosmic rays and high-energy solar particles interact with elements in the surface rocks and soil. After only a month of observations, the science team was able to generate a map showing the abundance of hydrogen in the uppermost metre of the planet’s surface. The distribution of the hydrogen with latitude was found to be almost identical to the distribution of stable sub-surface ground ice predicted from previous modelling studies. This means that the hydrogen detected by the Odyssey instruments may exist in sub-surface deposits of frozen water. Although it is premature to estimate total abundances, models indicate that at least the uppermost metre or so of the surface poleward of 60° in each hemisphere contains 3050% by weight of water ice. These permafrost-like deposits are, in other words, a potentially massive reservoir of water (figure 6).
Missions on the horizon
The exciting new discoveries about Mars are about to be augmented by equally or even more exciting results from a small armada of five spacecraft that will become operational within the next few years. These missions include the first Mars launches by the Japanese and European space agencies.
The Japanese Nozomi spacecraft was launched in 1998. Forced to take a circuitous route to the planet because of an early launch-system problem, it will arrive at Mars in early 2004 and will make detailed studies of how the solar wind interacts with its upper atmosphere. These observations will provide direct data on the rate at which various gases escape from the top of the Martian atmosphere.
The European Space Agency, meanwhile, will launch the Mars Express orbiter in the middle of this year. When it arrives at Mars in December, it will begin a series of comprehensive remote-sensing observations using a combination of high-resolution imaging, and ultraviolet, visible and near-infrared spectroscopy. On board will also be a radar/altimeter system that is designed to probe the structure and composition of the shallow sub-surface. The latter investigation will be the first attempt at orbital radar sounding of the planet. Indeed, if Mars Odyssey really does detect shallow sub-surface ice, then the Mars Express radar results are likely to excite scientists and the public alike.
The Mars Express orbiter will also deploy a small lander, called Beagle 2, down onto the surface of the planet in late December 2003. Beagle 2 — designed and operated by a team led by Colin Pillinger of the Open University in the UK — is a 30 kg stationary lander that will study the composition of materials on the surface of Mars and search for evidence of past or present organic chemical activity (see “One man’s mission to Mars” Physics World December 2002 pp1011). Compared with all previous planetary landers, Beagle 2 will have a much bigger proportion of scientific instruments. Although some astronomers worry that this may increase the risk of it not landing safely, the scientific pay-off could be substantial if the mission succeeds.
And finally, NASA will launch twin long-range rovers to Mars in mid-2003, in an endeavour called the Mars Exploration Rover (MER) mission. These rovers will land at different places on the planet in January 2004 and will each operate for at least 90 days on the surface. The 180 kg rovers can travel up to 100 m per day, and each carries a suite of nine cameras, three spectrometers and a tool for scraping and cleaning rock surfaces. MER aims to determine the composition and geological history of sites on Mars where conditions may once have been favourable to life. The landing-site selection process is nearly complete, and top candidates include a putative ancient crater lake and a region that is thought to contain minerals that may have been deposited by water. NASA wants to wait until the last minute before deciding which sites to aim for, so that it can take into account as much new information from MGS and Odyssey as possible (figure 7).
The future of Mars exploration
This is a tremendously exciting time for Martian exploration. While missions beyond Nozomi, Mars Express, Beagle 2 and MER are still only in the initial planning stages, the prospects over the next decade and beyond look excellent. NASA wants to launch the Mars Reconnaissance Orbiter in 2005, the French space agency is considering a science orbiter, while the Italian Space Agency (ASI) is planning a telecommunications orbiter. NASA and the European Space Agency are considering small surface missions called “Scout” and “Netlander”, respectively, both of which will arrive at the planet in 2007. NASA and the ASI, meanwhile, are considering a joint radar-mapping orbiter for 2009, and there are tentative plans for a multinational orbiter or lander that could start returning the first samples of Martian soil to Earth by 2011. Many of these plans are, however, still uncertain and the details will depend on the scientific results from the current suite of Mars missions.
Looking beyond these robotic missions, most scientists feel that human exploration of Mars will almost certainly be required to advance our knowledge of the planet’s possibly Earth-like past. However, there are substantial technological, political and financial hurdles to overcome before such human missions can begin. Travelling for several years in space would be physiologically and psychologically challenging, and there would be many practical difficulties in ensuring that the crew are safe and have enough food, water and oxygen to survive. Descending onto Mars and blasting back off the surface would be highly risky, while funding and managing a large international mission would be difficult in an era of short-term political attention spans. Despite these huge hurdles, many astronomers feel that the human exploration of Mars is the inevitable next step in the advancement and evolution of our species. What is less certain is whether those first dusty footsteps will be taken 20 years from now or 50.
Before then, however, we can look forward to further spectacular images and a wealth of additional scientific data from the current Martian missions. And as the upcoming missions begin their long interplanetary voyages to the red planet, astronomers using telescopes back here — both on and above the Earth — will also be making observations of Mars. We can expect wonderful high-resolution images this August, when our planet and Mars will be closer than they ever have been since the 1920s. In fact, it will not be until 2287 that Mars will be as close again. By then, however, our descendants may be looking back fondly through their own Martian telescopes at the bright blue world that they once called home.