Barry Sanders explains how state-of-the-art animations are taking over the role of classic quantum-mechanical Gedanken experiments to help visualize the complexities and challenges of the new quantum technologies
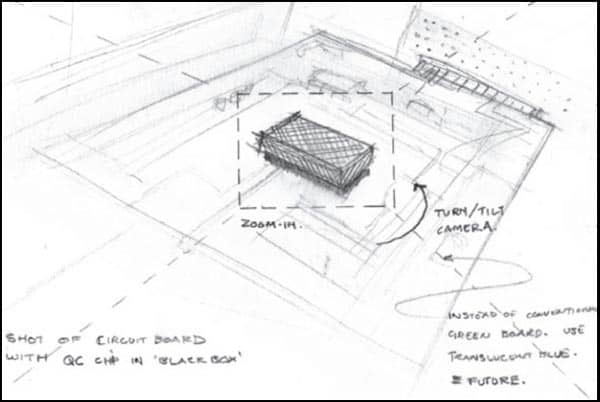
My interest in scientific visualization began when I was an undergraduate student at the University of Calgary and saw the film “Powers of 10” for the first time. The film begins and ends with the image of a man asleep at a picnic. In between the first and last scene, we zoom out from the picnic to the vast reaches of space, changing the distance scale by leaps of powers of 10. After reaching the size of the observable universe, at 1024 m, the view zooms back to the picnicker and into his hand, ultimately focusing down to the level of a single carbon nucleus, at the scale of 10–16 m.
Scenes from the film embedded themselves in my mind as vivid memories. I remember thinking about scaling in nature for weeks afterwards. For me, this unforgettable animation made clear the power of visualization in conveying abstract scientific concepts.
Visualization in physics has a long-standing tradition, particularly its application to quantum physics. In the early 20th century, the pioneers of quantum mechanics struggled even to explain their research to their colleagues and to convince them of its validity. Quantum concepts were strange and controversial, spurring hot debates between the likes of Niels Bohr and Albert Einstein. Scientific visualization, in the form of thought or “Gedanken” experiments, turned out to play a critical role in pushing quantum physics forward to maturity, as illustrated by Erwin Schrödinger’s influential semi-tragic story of the cat in a box. Werner Heisenberg’s “microscope” is another famous example.
Heisenberg’s “microscope” was first invoked in a paper from 1927 (Z. Phys. 43 172), to explain his eponymous uncertainty principle. Heisenberg considered the very simple set-up of a gamma-ray microscope that could accurately detect the position of an electron, at the expense of disturbing its momentum. Although unfeasible in practice, the choice of a gamma-ray microscopy was appropriate because only a short-wavelength electromagnetic field can resolve electronic motion within the atom.
Quantum short animations
Five years ago I returned to Calgary to form a new quantum-information group in the department of physics and astronomy to complement the existing quantum-computing group in the department of computer science. Early in the process, my group started attracting the interest of the wider university community and of funding agencies that were keen to learn about quantum information science and technology. My colleagues and I were faced with the long-standing challenge of communicating the essential elements of quantum physics to a more general audience. Inspired by the value of the Gedanken approach to explain difficult concepts, and enthralled by the rapidly developing power of animation, I started to believe that a combination of the two would be the best approach to explain the nature of the most challenging tasks in quantum information.
I began testing this approach with the help of my students. The first animation, done in collaboration with my assistant at the time, Rolf Horn, concerned quantum teleportation. We chose to make an animation of the famous teleportation of the polarization state of a single photon to another photon, as first demonstrated in 1997 by Anton Zeilinger and colleagues at the University of Innsbruck in Austria. The Innsbruck experiments were widely reported by the media, and an animation to explain this famous protocol and its experimental realization seemed like an excellent opportunity to try this new approach to the visualization of quantum-information technologies.
We first developed “amateur” versions of the animation, which we then showed to professional animators as a pitch for possible collaborations. The first of such collaborations came in 2003. After a few years creating animated quantum-information films, my portfolio was now such that it finally enabled me to obtain significant financial support for a state-of-the-art quantum-computing animation.
Quantum computing is a rapidly growing interdisciplinary endeavour dedicated to developing computers that will be able to solve tasks beyond the reach of classical computers, such as factorizing extremely large numbers. The stakes are high and the outcomes very promising, so funding is not as difficult to obtain as it is in other areas of science. With the help of researchers Andrew Greentree, Lloyd Hollenberg and Ashley Stephens from the University of Melbourne in Australia and Austin Fowler of the University of Waterloo in Canada, plus the skills of professional animators Andrew and Darran Edmundson of EDM Studio Inc. and audio expert Tim Kreger, we created the four-minute animation titled “Solid state quantum computer in silicon” in February 2007.
Quantum computer: the movie
In making the animation, we adapted the “Powers of 10” approach of zooming in and out to show a computer at different scales. We wanted to reveal how the computer would look to its user and how the computer’s components would appear if it were taken apart. We used a hybrid computer made of quantum and classical parts by introducing a “quantum chip” built into the “classical chip”. We were careful to show technical complexities such as the sophisticated electronics required for controlling the quantum chip plus the quantum bits (or qubits) and the quantum gates themselves. The multiscale approach uses zooming to show the interrelationships between these concepts.
Creating a visualization for science or technology requires managing the delicate balance between scientific accuracy and aesthetic appeal. Indeed, negotiations between scientists and animators can be more time-consuming and costly than the animation process itself. The whole team has to discuss and agree on visual representations and timing before the professional quality animations are done, otherwise valuable and time-consuming animations have to be discarded.
In quantum-information science, the qubit is the basic logical element and is analogous to the bit, or binary digit, of classical computers. The difference is that a bit can only assume the values of 0 and 1, but a qubit can assume the logical 0 state, the logical 1 state, or any superposition thereof. Physically, the qubit can be regarded as a spin-1/2 particle such as an electron, with an up state and a down state.
One of the challenges we faced involved depicting the “real” qubit and its environment while also showing its quantum-logical state. We chose to represent the qubit state as a point on a sphere, which is the standard in quantum information. The logical 0 state corresponds to the north pole and the logical 1 state to the south pole, with every other point on the sphere representing a superposition of these “polar states”.
For the type of silicon quantum computer in our animation, the physical realization of the qubit is the spin of the outermost electron of a phosphorous-31 atom that is embedded in a bulk silicon-28 medium. To make the qubit and its quantum state meaningful, we needed to show simultaneously the electron in the medium and the state of the electron spin.
The animation zooms into the bulk medium and shows the silicon lattice structure plus one phosphorous atom embedded in the medium. The phosphorous-31 atom looks like a sun in a lattice-like galaxy of silicon-28. To show the electron of the phosphorous atom, we used the standard portrayal of electron orbitals as clouds. The cloud is quite large and extends over the silicon lattice structure in every direction. The interaction between the electron cloud and the silicon lattice results in interference fringes in the cloud structure.
Depicting cloud interference is essential because of the importance of the overlap between the electron density and the nucleus. Magnetic fields extend throughout the quantum computer so that individual control of the electronic spin state, which serves as the qubit, requires precession of one atom’s electron while the others remain unchanged. This direct control is only possible by creating local electric fields via metal plates on the nearby surface of the silicon chip. The electric field distorts the electron cloud, as we show in our animation, and the distortion modifies the overlap of the electron cloud with the atomic nucleus. The visualization of the electron cloud and its distortion helps the viewer understand how individual electron spins can be controlled. The electron cloud conveys to viewers the distribution of the location of the electron orbiting the phosphorous-31, but the spin state has to be shown as well: quantum-information aspects have to be depicted alongside the physics. As discussed above, the spin state can be represented by a point on a sphere. We do this by placing a planet-like body near the phosphorous “sun” that shows its spin state.
The qubit is prepared and controlled by applying both global magnetic fields and local electric fields. We show the magnetic fields as broad faint lines in the solid-state medium and depict the precession of the electron’s spin state on the planet as a response to the application of these magnetic fields. The electric field is used to modify the “hyperfine splitting” of one atom so that its electronic spin qubit is individually addressable.
Meanwhile, we show the electric field as curved blue lines emanating from small metal structures on the surface. The electric field causes the electron cloud to change shape, and this shape change alters the cloud’s overlap with the phosphorous-31 nucleus, hence the hyperfine splitting. The precession of the electron spin is complicated by the presence of both a magnetic and an electric field, and we convey this complexity by showing the trajectory of the representation of the qubit spin on the “planetary sphere”.
Qubit control is just one step in quantum computing. In the animation we show two qubits and the application of the controlled-not, or exclusive-or, gate to the qubits, the readout of these qubits, and how the controlled-not gate would be performed using 28 qubits over a sequence of 45 steps, in a scene I call the “quantum error-correction dance”. For each step we need to make decisions about how to combine physical and informational entities in an aesthetic and meaningful way.
The power of visualization
Visualization of scientific knowledge is not easy or cheap, but it is rewarding and useful. Animated films are valuable tools for explaining difficult, abstract concepts such as quantum computing in the classroom. Unfortunately, at the time that it was created, our film was not widely released, but segments of it can now be viewed via an article in the December 2008 issue of New Journal of Physics (New J. Phys. 10 125005), and it is being used to great effect by the Australian Centre for Quantum Computer Technology partners, including in my classes at Calgary. The film has also been presented as part of quantum-information summer schools, including the Eighth Canadian Quantum Information Summer School in Montreal in 2008 and the International Summer School in Quantum Information Processing and Control at the National University of Ireland, Maynooth, in 2007. This animated film is an example of how visualization could be used in the future to help to effectively convey complicated scientific concepts and sophisticated emerging technologies.