Rising Force: the Magic of Magnetic Levitation
James Livingston
2011 Harvard University Press £20.95/$27.95hb 288pp
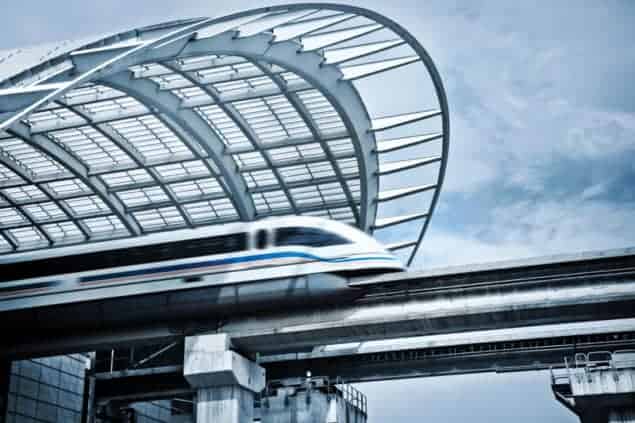
Let’s get the main message out straight away, before we get down to the details and maybe a few quibbles: in Rising Force, James Livingston has written an account of magnetic levitation that is a pleasure to read, informative, succinctly explained and scientifically accurate. This is a “popular-science” book, so the level of explanation is aimed at anyone who has vague memories of school physics. However, even if you remember much more, or are practising physics now, I guarantee that you will still learn something new.
Sometimes in a popular-science book, one finds that the author has oversimplified and distorted the truth. As a dyed-in-the-wool physicist, I could not resist the temptation to search for examples, but I was delighted to be disappointed in this case. Not only does Livingston know his stuff (he is now an emeritus lecturer at the Massachusetts Institute of Technology, and has spent a lifetime working in applied superconductivity and magnetism), he also knows how to get it across with a telling phrase. I savoured with pleasure his deft but accurate one-sentence explanation of how the always-downward force of gravity on Earth can nonetheless cause the upward motion of a hot-air balloon – the first physical means by which humans floated in the air.
Livingston is clearly well-rounded intellectually, and at the outset of the book he takes us on a magic carpet ride around the history of levitation. In addition to balloons, this account takes in the ancient stories of St Teresa of Avila, the magnetically supported island of Laputa in Gulliver’s Travels, Freudian dreams of flying, fake levitation by conjuring and (of course) the magic of Harry Potter, before the book gets down to the main business of magnetic levitation.
There are many reasons to be fascinated by magnetic levitation. One is an important practical consideration: by counteracting gravity you can remove a great deal of friction, and the consequences of this simple fact include 400 km h–1 trains already in passenger service in Shanghai. However, Livingston is equally captivated by newly acquired levitating toys, such as a spinning “levitron”. Livingston describes how he deduces the internal workings of his latest acquisition by plotting the fields around it with a gaussmeter – a great example of the importance of retaining a sense of “physics as play”.
Whether as a toy or practical application, however, all magnetic levitation has somehow to avoid the dictates of Earnshaw’s theorem, which states that it is impossible to set up an arrangement of static magnetic fields that will stably support another static magnet in empty space. Practical experience – whether as a child playing with magnets, or as an adult trying to make a friction-free bearing – strongly suggests that this theorem is true. Livingston makes extensive use of Earnshaw’s theorem, but he does not attempt to demonstrate why it must be true. I will now essay to do so in the style and at the level of the book, but in the context of electrostatics, to which it also applies. If you feel that my following demonstration is too complicated, then you may understand why Livingston did not include a proof!
Suppose we were able to levitate a single positively charged particle stably in empty space. “Complete stability” means that if the charged particle were to move away in any direction from its supposed point of equilibrium, there would have to be an electric field pointing back towards that point, to return the particle to its position of equilibrium. However, we know that electric field lines always end on charges and if all the field lines are directed towards the supposed equilibrium point, then there must be a negative charge there, and not empty space as we assumed. Hence, stable levitation is impossible and the particle must accelerate away from its supposed point of equilibrium in at least one direction. To make the proof more complete, I should add that the force of gravity can be represented as though it were an additional weak uniform electric field, so adding gravity to the picture does not change the conclusion.
Permanent magnets, of course, behave like dipoles, with north and south poles rigidly connected together. However, if we imagine such a pair of “opposite magnetic charges” moving together in any given direction, then because both the charges individually are unstable, the total force on the two charges is just as unstable. The other way that the dipole could change its position is by the magnet twisting around. This may or may not be unstable as well: anyone who has tried to balance one horizontal bar magnet above another will recognize that exchanging the positions of its north and south poles is one way that the top magnet can stop floating and stick to the bottom one. What is certainly true, though, is that whatever direction the dipole points in, it will still be unstable to sideways motion, so its ability to rotate does not help, and may hinder.
The one way that rotation can help make a magnet more stable is that if the magnet is set spinning at the right speed, then conservation of angular momentum will prevent it from turning over. Earnshaw’s theorem applies only to static magnets, so it has not prevented a certain Nate Tarler, of Florida, from keeping his toy “levitron” floating for nearly two years (with an external power supply to keep it spinning). In the book, you can find out much more about this toy, and the legal battles over its invention. Another “violation” of Earnshaw’s theorem described in the book will be more familiar to people working in condensed-matter physics: the levitation caused by the repulsion between a magnet and a superconductor. This phenomenon also escapes the famous theorem because it arises from induced diamagnetism, which acts as a feedback mechanism for stabilizing the magnet’s position, since the response of the superconductor depends on the position and strength of the magnet above it.
In a weak imitation of superconductors, a large number of materials, including water and alcohol, are slightly diamagnetic, so they are noticeably repelled by sufficiently large magnetic fields. This can lead to the “Moses effect”. An early report of this inspired André Geim to try levitating a frog in a high magnetic field, an achievement that won him an IgNobel prize (which was followed in 2010 by an unrelated Nobel prize for his work on graphene). However, I feel that he should also have levitated a ham sandwich, which would have led to the headline “Scientists show pigs can fly”!
Enough of this levity. Levitation by whatever means (and you will have to read the book to explore the multitude of others) has applications that range from nuclear proliferation, where it makes possible the enrichment of uranium in centrifuges, to microcircuit processing, where it facilitates dustless and frictionless movement of wafers. Livingston’s book discusses all of these, although one subject received rather too much coverage: his exhaustive list of levitating train projects that never took off was a little – well, exhausting.
My final comment is not so much about the book as the production values of Harvard University Press. It has brought out a hardback, but all the illustrations are a somewhat indistinct black and white, like those in a cheap paperback. If it had published Rising Force as an attractive “coffee-table” book, then maybe Livingston’s work would have reached a wider readership. As it is, readers will simply have to allow the brightness of its prose to illuminate its somewhat dull pictures.