Explosions and collapses are two of the latest effects to be observed in the most detailed study yet of the dynamics of Bose-Einstein condensates - the so-called fifth form of matter. Elizabeth Donley and colleagues at the University of Colorado and the National Institute of Standards and Technology are confident that their observations of ultracold rubidium atoms will produce new insights into the quantum world (E Donley et al 2001 Nature 412 295).
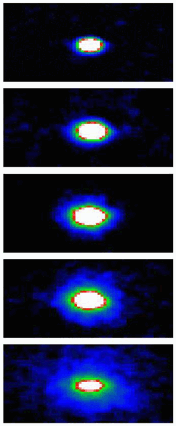
A Bose-Einstein condensate is a collection of gas atoms – cooled to a few millikelvin above absolute zero – that all occupy the same quantum state. This means that a single wave function describes the behaviour of all the atoms, and the quantum properties of the atoms can be seen in the macroscopic behaviour of the condensate.
Donley and co-workers used a magnetic field to control the attractive and repulsive forces between rubidium-85 atoms in a condensate. Such interatomic forces determine the properties – electrical, magnetic and mechanical – of all types of matter. The interactions between atoms in a Bose-Einstein condensate depend on the density of the condensate – which is typically ten thousand times less dense than air – and the ‘scattering length’. Rubidium-85 is unusual because its scattering length can be adjusted by an external magnetic field. A positive scattering length corresponds to repulsive forces between the atoms, while a negative value results in mutual attraction.
When Donley and colleagues suddenly made the attractive forces very strong, they found that the condensate imploded. But during the collapse, a proportion of the atoms exploded out of the condensate. Donley and colleagues found that these bursts of energetic atoms were ‘spin-polarized’, that is, they all had the same quantum mechanical ‘spin’.
Some of the atoms in the condensate did not take part in the collapse but remained in their original state for around a second after the implosion. The team calculated that the number of atoms in this state was related to the scattering length at which the implosion took place, and the initial number of atoms in the condensate. Donley’s team also forced jets of atoms from the imploding condensate by suddenly changing the scattering length before the collapse was complete.
Current theories of Bose-Einstein condensation cannot account for many of these observations. “We hope our work will generate new theoretical ideas to explain our data”, says team leader Carl Wieman. “This will provide a deeper understanding of Bose-Einstein condensation and quantum physics in general”.