Physicists have made yet another breakthrough in the field of Bose-Einstein condensation. Wolfgang Ketterle and colleagues at the Massachusetts Institute of Technology have succeeded in cooling a Bose condensate of sodium atoms down to below 500 pK. This is six times lower than the previous temperature record for Bose condensates. These ultracold samples could be important for spectroscopy, metrology and atomic optics applications (A Leanhardt et al. 2003 Science 301 1513).
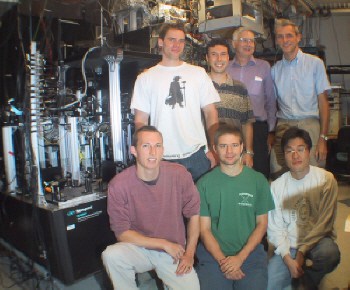
Bose-Einstein condensation occurs when a gas of atoms is cooled until the de Broglie wavelength of the atoms becomes comparable to the distance between them. The atoms then collapse into the same quantum ground state.
Researchers made the first Bose condensate in 1995 with rubidium atoms and have since created condensates from seven other elements, including sodium. Advances in cooling techniques mean that physicists can now routinely cool atoms to as low as a few nK. However, they have not yet managed to reach below 3 nK.
The MIT team first trapped several million sodium atoms in an ‘optical tweezer’. This focused laser beam induces an electric dipole moment in the atoms, which attracts them to the intense electric field of the laser’s focus. They reduced the power of the laser so that the most energetic atoms could escape and cool down the atoms that remained behind. This technique is called ‘evaporative cooling’.
Ketterle and co-workers then transferred the condensate to a special ‘gravito-magnetic’ trap that confines the atoms. The resulting partially condensed gas cloud contained about half a million atoms and had a temperature of about 30 nK. They then weakened the gravito-magnetic trap and this cooled the gas further in a process known as ‘adiabatic decompression’. The temperature of the condensate, at that point containing about 200 000 atoms, dropped below 3 nK.
“It is the same physics behind a pressure cooker,” explained team member Aaron Leanhardt. “If you compress a gas, that is make the confinement stronger, you heat it up. But if you allow the gas to expand by weakening the confinement you cool it down.”
The group then performed another cycle of evaporative cooling to lower the temperature of the cloud even more. This reduced the number of atoms in the condensate to only 30 000 and resulted in a temperature of below 1 nK. The lowest temperature the team measured was 450 pK.
The researchers point out that they could achieve lower temperatures by further weakening the trap and reducing the number of atoms, but admit that this would be “challenging and technically difficult”. As well as practical applications –such as improving atomic clocks and interferometers – they are interested in studying how these ultracold atoms interact with room temperature surfaces. Theory predicts that they should undergo quantum reflection.