Chemical physicists at the California Institute of Technology have developed a new approach to nuclear magnetic resonance (NMR). The new method - known as better observation of magnetisation, enhanced resolution and no gradient (BOOMERANG) - can image liquids as well as solids, and could lead to the development of portable NMR instruments that might be used at the micron scale and below (L A Madsen et al. 2004 Proc. Nat. Acad. Sci. 101 12804).
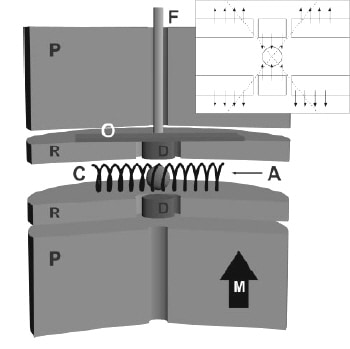
In conventional NMR, a sample is placed in an external magnetic field, which aligns the spins of any nuclei with magnetic moments in the sample. Radio-frequency (RF) pulses are then sent into the sample, causing the spins to point in different directions. After a certain time the spins return to their equilibrium positions and re-align with the magnetic field, re-emitting RF waves as they do so. Measuring this radiation shows how abundant these nuclei are in the sample.
BOOMERANG, on the other hand, measures a force and not a RF signal. Moreover, it uses a homogenous magnetic field, whereas many NMR methods rely on magnetic fields that have a gradient. Imaging liquids with conventional NMR techniques is difficult because gradient fields can cause molecules with a nuclear magnetic moment to diffuse through the liquid. The new method, devised by Daniel Weitekamp and colleagues at Caltech, reduces de-phasing caused by this diffusion to almost zero.
The uniform field is produced by two millimetre-sized permanent magnets, between which the sample is placed (see figure). When pulses of radio waves are applied, nuclear spins in the sample interact with magnet moments in one of the ferromagnets. This causes the magnet in question to move slightly and this force – the signal – is then detected by a fibre-optic interferometer. The Caltech team used its device to successfully distinguish signals from hydrogen and fluorine-19 nuclei in a millimetre-sized drop of liquid fluoroacetontrile (CH2FCN).
The BOOMERANG technique can easily be extended to the micron and nanometre scales, says Weitekamp, which means it could be used for imaging single biological cells, microfabricated structures and other samples with sub-millimetre features. Weitekamp and co-workers are now collaborating with the NASA Jet Propulsion Lab to extend the method for use on 60-micron samples. The group is also working on new designs to further improve the sensitivity of the device.