Physicists have found conclusive evidence for superfluidity - flow without resistance - in an ultracold Fermi gas. Although indirect evidence for superfluidity in Fermi gases has been seen before, low-temperature physicists have been searching for definitive evidence in the shape of quantized vortices in a rotating gas. Now Wolfgang Ketterle and colleagues at the Massachusetts Institute of Technology (MIT) have observed these vortices in a gas of lithium-6 atoms (Nature 435 1047). The results could shed new light on systems as diverse as high-temperature superconductors and the quark-gluon plasma.
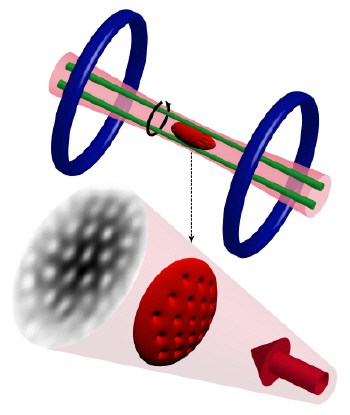
All atoms are either bosons or fermions depending on the value of their intrinsic angular momentum or “spin”, and the difference between the two becomes clear at ultracold temperatures. Bosonic atoms have integer spins in quantum units and can collapse into the same quantum ground state in a process known as Bose-Einstein condensation (BEC). This process is at the heart of both superconductivity – the flow of electric current without resistance – and superfluidity.
Fermions, on the other hand, have half-integer spins and obey the Pauli exclusion principle. This means that two fermionic atoms cannot occupy the same quantum state. However, they can bind together to form a bosonic molecule that can then undergo condensation. Similarly, electrons – which are fermions – can form “Cooper pairs” and undergo condensation in the Bardeen-Cooper-Schrieffer (BCS) theory of superconductivity.
It is the possibility of reproducing this Cooper-pairing process in a Fermi gas, and possibly learning more about the mysterious pairing mechanism that underpins high-temperature superconductivity, that has led to intense interest in these systems.
Ketterle and co-workers started with a lithium-6 gas that had been cooled to about 50 nanokelvin and then applied a magnetic field to change the strength of the interactions between the atoms. For certain field strengths the atoms formed molecules that subsequently condensed to form a molecular BEC.
Next, the MIT team increased the strength of the magnetic field to convert the molecular condensate into a Fermi gas with strong interactions between the atoms. Finally, they used a green laser beam as a “spoon” to vigorously stir this gas and make it rotate.
In contrast to a normal fluid such as water, a superfluid can only rotate by forming a regular array of quantized vortices, each of which carries part of the total angular momentum of the superfluid. In addition to expelling atoms from their centres to leave a string-like hollow core, the vortices also repel each other to form a regular lattice pattern.
“When we observed the beautiful array of vortices, we instantly knew we had created a new form of matter – a high-temperature superfluid,” says Martin Zwierlein, lead author of the paper. “It might sound confusing to call it a high-temperature superfluid, but scaled to the density of electrons in a metal, the transition from a normal gas to a superfluid would occur well above room temperature.”
Zwierlein also points out that the size of the Cooper pairs in a superconducting material is fixed, whereas the properties of the atom pairs in a superfluid Fermi gas can be changed by simply changing the magnetic field. “Thanks to this unique level control,” says Zwierlein, “superfluid Fermi gases can serve as model systems for high-temperature superconductors and even more exotic forms of matter such as neutron stars or the matter that existed in the early stages of the universe.”