Physicists in the Netherlands have created artificial crystals made of colloidal particles that could be used to explore the behaviour of ordinary ionic crystals. The team discovered a number of new crystal structures with the system, which could also be useful for applications such as "electronic-ink" (Nature 437 235).
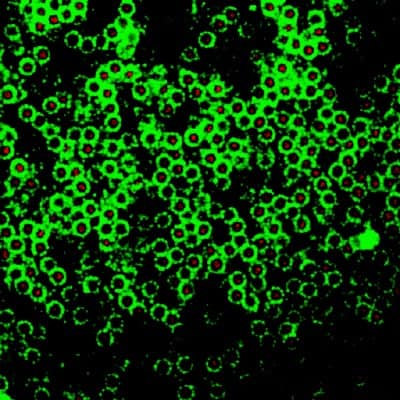
Scientists routinely use colloid suspensions to model solids and liquids, such as the way they melt and freeze. They are able to follow the motion of the individual colloidal particles during these processes because they are about 1000 times bigger than atoms and molecules. It is also possible to change and control the interactions between the colloid particles to a certain extent. However, it has been difficult to model long-range or ionic interactions until now because the attractive interactions between oppositely charged particles have always been too strong, which has lead to the formation of irregular clusters of particles called aggregates (figure 1).
Mirjam Leunissen, Christina Christova and colleagues at Utrecht University have now overcome this problem. They started by making colloidal spheres with positive and negative charges, which they coloured differently with dyes. Then they mixed the spheres in a solution and followed their behaviour with a confocal microscope. They were able to tune the charge on the spheres by adding a small amount of a salt (tetrabutylammonium chloride) to the mixture: keeping the charges sufficiently low ensured that the particles did not form aggregates (figure 2).
As well as being able to study processes like crystallization and glass formation, the Dutch researchers were also able to create a number of unusual new structures that they had predicted on the basis of computer simulations (figure 3). “This is remarkable because we have only investigated two different sized particles so far,” says Leunissen.
In an ionic crystal, for instance, the total charge on the negative ions tends to cancel out the total charge on the positive ions. In some of the structures created by the Utrecht group, however, the total charges on the particles do not cancel each other out, although neutrality is maintained by the liquid they are suspended in.
The Utrecht group has also shown that it can melt the crystals in a controlled way by applying a low electric field — a process that would require unfeasibly high fields in a real atomic crystal. This is possible because the density of colloid crystals is a billion times smaller.
The team also observed for the first time that colloid particles moving quickly in the same direction group together to form stripes, again confirming the predictions of theorists. The new structures could be useful for display applications, such as e-ink, because they are sensitive to electric fields (figure 4).