In 1956, physicists Robert Hanbury Brown and Richard Twiss showed that the intensities of light from two different points on a random light source such as a star are correlated. However, this correlation vanishes when a coherent light source such as a laser is viewed. Now, physicists in France and Portugal have discovered the equivalent effect in ultracold quantum gases, demonstrating that, at sufficiently low temperatures, identical atoms are more likely to be observed close together than far apart. The effect, or its absence, could therefore help researchers determine the state of quantum gases (Sciencexpress 1118024).
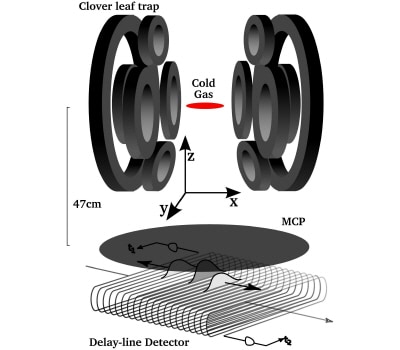
The first observation of the Hanbury Brown Twiss effect in an ultracold gas was made by researchers from the University of Tokyo in 1996 with neon atoms, but the team was unable to study the effect in any detail. Now, Chris Westbrook and colleagues of the University Paris-Sud and co-workers at the University of Minho have developed a detector that can identify the arrival of single atoms of ultracold helium on a time scale of nanoseconds and distances less than 200 microns. This allows the team to detect any small atomic correlations between atoms that might be present.
Although such detectors are routinely used to study atomic and ionic collisions, they have never been used for Bose-Einstein condensates. First produced in 1995, a Bose-Einstein condensate (BEC) is a collection of atoms that has been cooled to such low temperatures that all the atoms collapse into the same quantum state. Most BECs have been formed from alkali atoms such as sodium and rubidium, but detecting individual atoms is easier when metastable helium atoms — from which Westbrook’s team and other groups in France first produced BECs in 2001 — are used.
Westbrook and co-workers found strong correlations in the ultracold helium gas when it was above the transition temperature for a BEC to form, but the correlations disappeared when the gas was cooled below the transition temperature. According to Westbrook, this is because the quantum interference effect that normally increases the probability of finding two events close together is not present in a BEC.
“Our technique will be a great tool for studying BECs and other quantum gas systems, especially near a phase transition,” says Westbrook. The team now plans to investigate phase transitions to Mott insulators and atom correlations due to Feshbach resonances, which have been central in allowing researchers to produce molecular and fermionic condensates in recent months.