Quantum computers and blades of grass have more in common than you might think. Physicists in the US have shown that electrons involved in photosynthesis reactions "sample" different energy-level routes in much the same way quantum-computer algorithms can -- at least in theory -- quickly search through unsorted databases. The researchers claim that the discovery could explain how photosynthesis can proceed at efficiencies unparalleled in manmade solar cells (Nature 446 782).
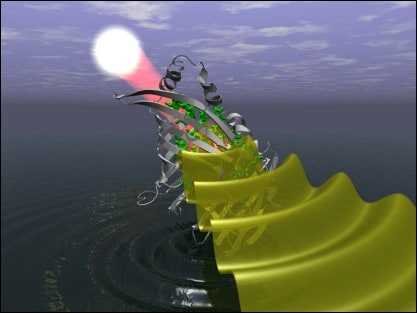
Arguably the most important chemical reaction on Earth, photosynthesis allows plants to harness the Sun’s energy by converting carbon dioxide and water into energy-rich carbohydrates. For the most part this takes place in chlorophyll molecules, which are arranged such that neighbouring molecules have different energy levels. When light shines on one of these molecules, an electron is momentarily excited before passing its energy over to a nearby molecule with a slightly lower energy level. In this way energy can flow “downhill” from energy level to energy level until it reaches the crucial “reaction centre” where the actual photosynthesis occurs.
Scientists had assumed that the energy moves downhill in a “random walk”, which is essentially an incoherent “hopping” between energy levels. But this mechanism doesn’t explain how solar energy is transferred so quickly to a reaction centre, which allows photosynthesis to proceed with efficiencies of 95% or more. Gregory Engel and colleagues at the University of California in Berkeley, however, may now have the answer – and it’s all to do with quantum mechanics.
The US team performed 2D electronic spectroscopy to map the electron-energy levels of a certain strain of chlorophyll. They discovered regular variations of signal that sustained for hundreds of femtoseconds, which the physicists interpreted as “quantum beats” coherently linking all the energy levels together. Engel told Physics Web that this means the excitation can find the optimal route to the reaction centre without wasting energy through random hopping. “In effect, the excitation can ‘feel’ these many states at once without having to visit them individually,” he said.
It turns out that the computer scientist Lov Grover stumbled across an analogous method in 1997 by developing “Grover’s algorithm”, which he proved to be the fastest possible search of an unsorted database in quantum computation. “In the [photosynthesis] case, the energy searches for the path it needs to follow to get to where it’s useful,” explained Roseanne Sension at the University of Michigan.
Some may have qualms over Engel’s conclusions, however, because the experiment was performed at a temperature of just 77 K. The physicists chose this to exaggerate the behaviour of the electrons so that the experiment would be easier to demonstrate. But they insist that the energy transfer process would remain the same at more hospitable temperatures. “I’m not convinced it will,” said Sension. “Things are more rigid at low temperatures, so there is less [chance] that it will lead to decoherence. But it remains to be seen.”