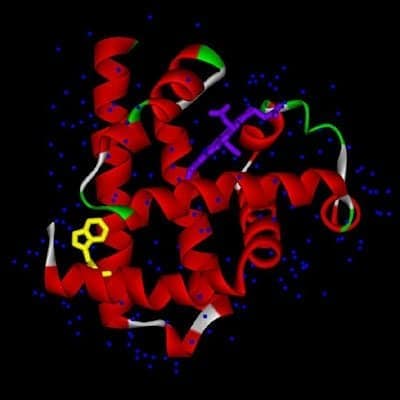
Living organisms contain both proteins and water and the complex interactions between the two are thought to be the driving force behind many biological processes.
Now, biophysicists in the US have discovered that a protein called myoglobin can coordinate the motion of surrounding water molecules, slowing them down significantly – perhaps to allow certain interactions to occur (PNAS 104 18461). The team has also shown that the motion of these water molecules can be associated with the shape and function of the protein – information that could improve computer simulations of protein dynamics and lead to a better understanding of diseases like Alzheimer’s and Parkinson’s, which involve drastic protein shape changes.
Proteins are long strings of amino acids that fold into compact structures. They are found in all living organisms, where they perform myriad tasks from chopping food into its molecular constituents to contracting the muscles that allow us to move. In order to do their jobs, most proteins must stay folded but also change their shapes in controlled ways – despite being jostled relentlessly by the rapid thermal motion of surrounding water molecules.
Fleeting interactions
Biophysicists have long suspected that the interactions between proteins and surrounding water molecules – a process called protein hydration – play important roles in protein folding and function. However, these interactions had been very difficult to study because they are so fleeting – sometimes lasting less than a billionth of a second.
Over the past few years Dongping Zhong and colleagues at the Ohio State University have developed a way to study protein hydration using ultrashort pulses of laser light. Their technique involves the amino acid tryptophan, which occurs naturally in proteins. When tryptophan is excited by a laser pulse, it emits light with properties that depend on how the tryptophan is interacting with nearby water molecules.
The team prepared proteins called myoglobins (proteins that carry oxygen in muscles) with tryptophans at known locations along the protein strands. The team then fired 90 fs (9*10-14 s) pulses of ultraviolet light at the proteins. By observing the light emitted from the tryptophans, they discovered that water next to the proteins moves in two very distinct ways.
Collective motion
One mode of motion is the “slow” collective movement of a layer of only water three molecules thick that surrounds the protein. This “slow” motion occurred on timescales of 20-200 ps (20-200 * 10-12 s) and was distinct from the “fast” (1-8 ps) movement of individual water molecules that the team detected at greater distances from the protein. Both types of movement are significantly slower than the motion of water molecules in the absence of proteins.
Zhong and colleagues also discovered that the value of the slow timescale had a strong association with changes in certain local surface properties of the myoglobin including electrical charge and flexibility. Both of these properties are known to be important factors that determine how a protein changes its shape.
Although the exact speeds of the “fast” and “slow” motions differed over the protein’s surface, the two types of motion were present at all tryptophan locations and even persisted when the protein’s structure was disrupted with a mild acid. Zhong told physicsworld.com that this means that that the two modes are likely to be a general property of all proteins. “We are now testing other types of proteins with different structures,” he said.
While water has a simple molecular structure, biophysicists have struggled to understand how it interacts with proteins on the lengths and timescales investigated at Ohio State, and instead have relied mostly on computer simulations. According to Zhong, his team’s discovery of a slow collective motion of water puts into question the validity of these computer models – particularly the timescales used. “We are pretty confident that the simulations need to change”, he said.