Light usually travels in a straight line – but not when it crosses a gap between two plates that are less than a wavelength apart. Physicists have known about this strange behaviour for some time, but it has proven very difficult to predict exactly what path the light will follow. Now, however, researchers in the US have created a computer model that does just that, and it could be a boon to those trying to harness the unique optical properties of tiny gaps to make more sensitive microscopes and more efficient photovoltaic cells Appl. Phys. Lett. 91 153101.
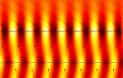
When light is radiated between two surfaces separated by less than a wavelength, there is not enough room for the light to cross the gap as a propagating wave. Instead it bridges the gap via “evanescent” waves, which are standing waves that only exist about a wavelength or less from a surface. While physicists are starting to exploit such waves in nano-optical devices such as superlenses, exactly how light behaves in tiny gaps is very difficult to predict using electromagnetic theory.
Now, Zhuomin Zhang and colleagues at the Georgia Institute of Technology have come up with a new way to predict the behaviour of infrared light in such gaps. Instead of focussing on the light itself, they modelled how evanescent waves transfer heat across a 100 nm vacuum gap between two plates of silicon carbide. This involved calculating the “Poynting vector”, which describes the flow of electromagnetic energy.
Their simulations show that instead of travelling in a straight line, the light snakes its way across the gap. The team believe that this new ability to visualize how light travels across tiny gaps should help physicists make important decisions when designing nano-optical devices — such as what shape the plates should be and how far they should be separated.
According to Zhang, the simulations could help researchers create nano-optical structures that are extremely good at absorbing infrared radiation. Such structures could be used to make very efficient thermophotovoltaic (TVP) cells, which convert infrared radiation into electricity. TVP cells could someday be used to generate electricity from the waste heat of industrial processes.
The simulations also provide a vivid illustration of the negative refraction of light at both of the vacuum/silicon carbide interfaces. Negative refraction occurs when light travelling from one medium to another bends in the direction opposite to the direction normally associated with refraction. Physicists have already taken advantage of this effect in “superlenses” made from slabs of silicon carbide that are able to take images of tiny structures that are much smaller than the wavelength of light used.