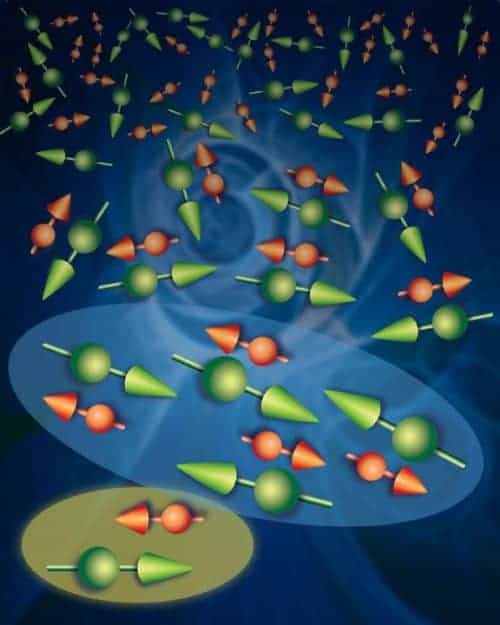
An international team of physicists has turned a common semiconductor material into an unconventional type of metal called a “non-Landau–Fermi liquid”.
While this is not the first such metal to be made, the team claims that it is the first that can be described by a simple theoretical model. This could help physicists understand more complicated non-Landau–Fermi liquids such as high-temperature superconductors and also could lead to new ways of controlling spin-polarized electrons.
One of the most remarkable properties of individual conduction electrons in most metals is that they appear to go about their business oblivious to the fact that they are crammed into a solid lattice along with 1022 other electrons and ions. Instead of ricocheting from one collision to the next, the electrons appear to flow smoothly through a metal much like a fluid through a pipe.
Non-interacting electrons
This behaviour was first explained in 1956 by the Soviet physicist Lev Landau, who argued that the effects of the interactions between the electrons could be accounted for by describing the system as a collection of non-interacting electrons, called “quasiparticles”, each with an “effective mass” greater than the mass of a free electron.
The Landau-Fermi model relies on the idea of “screening” — that the electrostatic forces within a cloud of negatively charged electrons are cancelled exactly by the positively charged ions. Similarly, the interactions between the spin magnetic moments of electrons and ions are also screened.
Landau–Fermi liquid theory has been extremely successful at describing the behaviour of systems as varied as metals and liquid helium. However, physicists have discovered a small but growing number of materials in which electrons move freely, but cannot be described by the Landau–Fermi liquid model. The most notable being the high-temperature cuprate superconductors, which have proven very difficult to understand.
Researchers are therefore keen to understand the properties of such “non-Fermi liquids”, but have yet to find a simple system that can be studied both experimentally and theoretically.
Simple theory
Now Gabriel Aeppli at the London Centre for Nanotechnology and University College London, and colleagues at the National University of Lesotho, Louisiana State University and Bell Laboratories in New Jersey, have discovered that doped iron silicide (FeSi) can be a non-Landau–Fermi liquid. What’s more, they say that the behaviour can be predicted by a simple theory (Nature 454 976).
FeSi is a small gap semiconductor that has been widely studied because its optical and electronic characteristics can be easily altered by doping it with impurities such as aluminium and cobalt . While this creates disorder in the material, the doped FeSi samples previously studied were Landau-Fermi liquids.
In this new work Aeppli and colleagues doped FeSi with manganese atoms, contributing an ionic core with a spin magnetic moment of one. However, each manganese impurity also creates a positively-charged “hole”, which is a quasiparticle with a spin magnetic moment of 1/2. This means that the spin magnetic moments of the impurities cannot be fully screened by the spins of the holes.
Quantum ambiguity
At very low temperatures of a few Kelvin, quantum mechanics dictates that some manganese impurities will be fully screened, while the rest are not screened at all. According to Aeppli, this ambiguity as to which impurities are being screened at a particular time means that the quasiparticles in the material cannot endure on time scales long enough to constitute a Landau-Fermi liquid.
The team confirmed this by measuring the electrical conductivity of the doped material as a function of temperature. The conductivity of a disordered Landau-Fermi liquid always varies as the square-root of the temperature, but that was not the case for the manganese-doped FeSi. The team also applied a relatively weak magnetic field to the sample – which caused the material to conduct like a disordered Landau-Fermi liquid. The team believes that the manganese spins tend to align themselves in the direction of the magnetic field, thus removing the quantum-mechanical ambiguity.
According to Aeppli, it should be possible to take advantage of this effect in electronic devices in which the electrical current flowing through a junction can be switched between a Landau–Fermi liquid and non-Landau–Fermi liquid. Such devices could find use in spintronics — electronic circuits that use both the charge and spin of electrons to process information.
This quantum ambiguity is an example of quantum entanglement – a process that is vital to the operation of quantum computers. As a result Aeppli believe that this effect could be used control the translation of quantum information from stationary electrons found near the cores of ions to moving electrons.