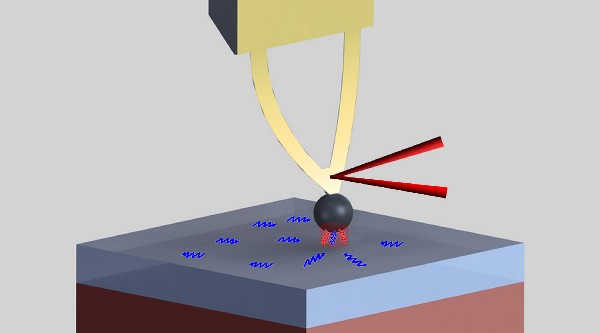
Long-held suspicions that Planck’s law is violated at microscopic length scales now have the backing of experimental evidence.
Scientists at the Massachusetts Institute of Technology (MIT) and Columbia University have shown that the law, which relates the radiation produced by an object to its temperature, under-estimates by up to three orders of magnitude the heat radiated when two objects are separated by just 30 nm.
These radiative heat-transfer measurements — the first on this length sale — were carried out by adding a gold layer to the cantilever of an atomic force microscope (AFM) so that it bends according to the amount of heat transferred.
Planck’s doubts
First formulated in 1900, Max Planck always suspected that his eponymous law was not valid at length scales comparable to the wavelength of thermal radiation, a regime known as the near-field. Calculations of radiation at these microscopic distances followed in the 1950s, but experimental verification proved elusive — until now.
Testing Planck’s law at the near-field requires holding two objects very close together, but preventing them from ever touching. “We tried for many years doing it with parallel plates,” says MIT’s Gang Cheng. But with that method, the smallest sustainable separations were a micron or more.
Switching to an AFM-based approach was the key to success. By attaching a small round glass bead to the apex of the cantilever, the researchers realized separations of tens of nanometers. The advantage of using a spherical object is that it is far easier to maintain a distance between single point and a surface, than between two surfaces.
Mitigating conduction
Cheng’s team measured heat-transfer rates between the glass bead and three different types of substrate: glass, doped-silicon and gold. Experiments were performed under vacuum to eliminate significant heat conduction across the air gap.
The researchers hit the gold layer of the cantilever with a 650 nm laser, which heated the sphere before it radiated and cooled. The extent of this heat loss was revealed by the subsequent bending of the cantilever, which is caused by the differences in the thermal expansion coefficients of the two materials.
The highest heat-transfer coefficient was found to occur between a glass bead and a glass substrate. These polar dielectric materials have surface phonon polaritons — which are created by resonant coupling between the electromagnetic field and optical phonons in polar dielectrics — and they strongly enhance the near-field radiation.
Improved recording heads
The efforts of Chen and his co-workers are not just of fundamental interest: they could also improve the thermal design of today’s magnetic heads in hard disc drives, which are separated from the discs by 5–7 nm. A resistor in the head causes it to heat up, and this recent work could allow engineers to manage this heat, or even use it to control the gap.
“There are also companies looking into heat-assisted magnetic recording technology,” says Chen. One option for increasing data storage density is to shrink the size of the data bits, but if they are too small data loss occurs due to magnetic domain fluctuations. “People have developed much harder magnets to prevent such fluctuations, but it is difficult to write on hard magnets. But using local heating, one can write the data,” he added.
The next goal for the researchers is to repeat their measurements at shorter separations. “Surface roughness is a big challenge,” says Chen, “and other problems are that of mechanical stability and cleanliness of surfaces — both are not easy to quantify.”
This research was published in Nano Letters.