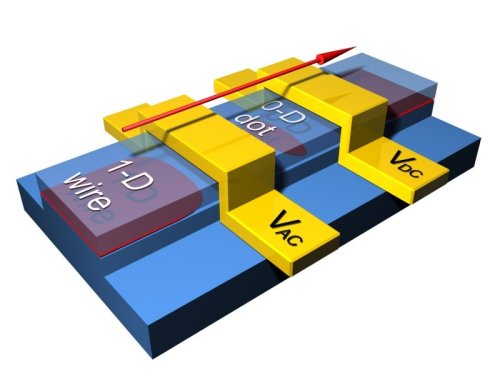
A device that emits exactly one spin-polarized electron every billionth of a second has been unveiled by physicists in Germany. Based on a tiny piece of semiconductor called a quantum dot, the device is one of the fastest single electron pumps ever built.
The researchers believe that the device could, with some improvements, be used as a very precise source of electrical current that would allow physicists to redefine SI units in terms of fundamental quantities such as the charge of the electron — a discipline called quantum metrology.
On a more practical level, as the spins of the emitted electrons appear to all point in the same direction, the device could be used as a source of spin-polarized electrons in “spintronic” devices, which exploit both the spin and charge of the electron. In principle, this would allow such devices to operate at gigahertz clock speeds.
Tunnelling devices
The new device has been built by physicists at the PTB standards lab in Braunschweig, Germany. They are among a number of groups at standards labs around the world developing precise single-electron current sources. Many of these sources take advantage of the quantum mechanical effect of tunnelling — whereby an electron has a probability of spontaneously crossing an insulating barrier between two tiny pieces of metal (Applied Physics Letters 94 012106).
Early devices employed a series of tiny metal pieces and barriers. However, tunnelling takes a finite amount of time, which means that there is a gap of about one ten millionth of a second between the emission of successive electrons. In other words the source operates at 10 MHz, and this relatively low frequency results in an electrical current that is too small be of any practical use as a precise current source for quantum metrology.
Much faster single electron pumps that operate at gigahertz frequencies have been made using surface acoustic waves (SAWs) on a semiconductor. These are high frequency sound waves in a semiconductor that drive single electrons across and insulating barrier. However, these are much less precise than those based on tunnelling and therefore are not likely to be of much use for quantum metrology.
In 2007 an international team of researchers including Mark Blumenthal at Cambridge University, Bernd Kaestner at the PTB national metrology lab in Germany and J T Janssen at the UK’s National Physical Laboratory worked out a new way to make a much faster single electron source based on oscillating tunnelling barriers.
Comprising a tiny piece of semiconductor called a quantum dot, the device was initially operated at frequencies up to about 3 GHz. However it was nowhere near precise enough for quantum metrology because it failed to spit out exactly one electron about once in every 10,000 cycles. To be of practical use, a GHz pump could only skip a beat about once every 10 million cycles.
In December last year, Janssen and colleagues in Cambridge and New York showed that the performance of such an electron pump could be improved greatly by placing it in a magnetic field as high as 3 T. Now, Kaestner along with Hans Schumacher and colleagues at PTB have boosted this magnetic field to about 10 T and discovered that the performance is improved even more. What’s more, at such a high field, the pumped electrons are almost certainly spin polarized — according to the researchers.
Oscillating voltage
The PTB device is based on a quantum dot 250 nm in diameter (see diagram). Opposite sides of the quantum dot are connected to two tiny metal wires (700 nm wide) via two thin insulating layers (about 100 nm thick). An electrode carrying an oscillating voltage is placed onto one insulating layer and an electrode carrying a constant voltage is placed onto the other insulating layer.
An electron can move from a wire to the dot by tunnelling through the insulating layer under the oscillating-voltage electrode. This is much more likely to occur at a certain point in the oscillation, when the tunnelling barrier is reduced by the applied voltage. After tunnelling has occurred, the quantum dot then has an extra electron, which then tunnels out into the other nanowire. Electrical repulsion between electrons means that only one electron can squeeze through the tunnel barrier at a time.
Schumacher told physicsworld.com that such devices can operate at gigahertz frequencies because the applied voltages reduce the tunnelling barriers, allowing tunelling to occur much faster than in the metal-insulator devices.
The team found that by applying a high magnetic field to the dot, the precision of the pump went from about one in 10,000 to about one in one million. However, it is not clear exactly why the magnetic field has such a significant boost on the precision.
Schumacher believes that the magnetic field changes how the electron tunnels through the insulator. The electron is deflected by the magnetic field and therefore follows longer curved trajectories through the barriers — which could make it more likely that exactly one electron tunnels in and out per cycle. Janssen adds that the magnetic field also has the effect of confining the electrons into a smaller region of the quantum dot — which sharpens the divisions between electron energy levels, again making it more likely that exactly one electron moves in an out.
‘Interesting’ technology
Jukka Pekola, who studies single electron sources at the Helsinki University of Technology, described the source as “interesting” because the magnetic field gives physicists another parameter to tune its performance — and also because the electrons appear to be spin polarized.
The PTB team is now trying to further boost the precision of the source to about one in 100 million by trying to find the optimum magnetic field, device shape, operating frequency and waveform of the oscillating voltage.
While the team have not actually measured the spin polarization of the electrons, Schumacher is confident that the 10 T field aligns all the spins in the same direction. But just to be sure, the team plans to measure the spin polarization at sometime in the future.
Schumacher adds that the source can easily be adjusted to produce two spin-polarized electrons at a time. Because the pair is created in the same quantum dot, they would be entangled and therefore could be used — at least in principle — in a quantum computer.