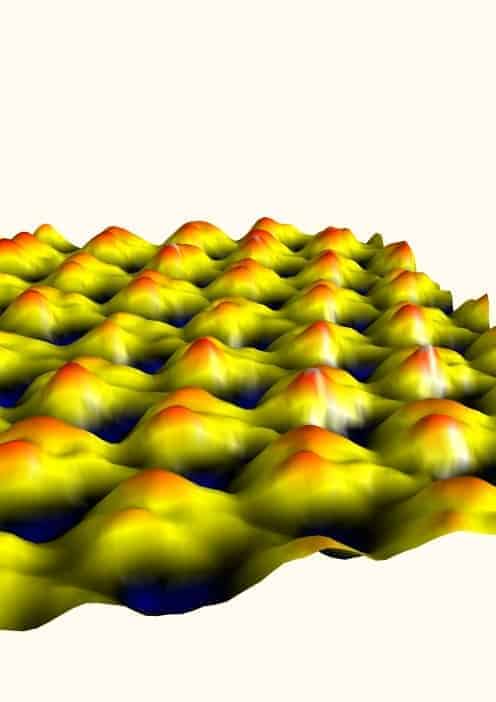
Researchers in the US have used a modified atomic force microscope to find the flexible regions of a protein. The motions of proteins can play important roles in their biological function and therefore understanding which parts of the molecule can easily bend — and how this suppleness is affected by the presence of other molecules — could help in the development of new drugs.
Proteins are chains of amino acids that are involved in just about every biological process. They are essentially nanoscale mechanical devices with flexible moving parts, as well as more rigid components. According to Ozgur Sahin, who led the study at Harvard University’s Rowland Institute: “If one can identify flexible parts of proteins, one can design small molecules (drugs) to bind and alter their flexibility.”
Sahin and team have used their modified AFM to study the flexibility of bacteriorhodopsin — a protein used by some micro-organisms to convert light into electricity. The molecule is a distant relative of rhodopsin, which performs the role of detecting light in human eyes.
Tiny forces
The AFM itself works by measuring how the force between the sample and a tiny “tip” on a cantilever changes as the tip is moved over the surface of the sample. This allows the AFM to record images with extremely high spatial resolution on the nanometre scale.
The flexibility of a material can be measured by pushing the AFM cantilever against a sample — and measuring the deformation of the sample as a function of the applied force (the elastic modulus). However, this method has its drawbacks when used on tiny and fragile proteins. “If the cantilever is soft, the forces can be determined accurately, but the sample will not be deformed — rather, the cantilever will bend ” explains Sahin. Conversely, if the cantilever is stiff, the sample will be deformed, but it will be difficult to measure the forces.
The team addressed the challenge by designing a T-shaped cantilever that can both twist and bend. The tip is located at one end of the crossbeam and sensitive measurements of the forces are made from the twisting motion of the cantilever and deformation is determined from its bending.
The AFM was operating in vibrating mode, which means that the tip was oscillating rather than stationary. Successful measurements result from careful adjustments to resonant frequencies and spring constants of the bending and torsional modes of oscillation.
Flexible “switches”
Before being placed in the AFM, bacteriorhodopsin molecules were embedded in micrometer-sized cell membranes. The membranes were placed on a flat surface and the AFM was used to measure their elastic moduli. The measurements revealed that the region that contains the protein’s “electrical switch is about three times more flexible than the the rest of the structure.
Armed with the T-shaped cantilever, the team could perform a single force measurement in just 120 μs. By comparison, a standard AFM would take 1 s to perform the same measurement, and consequently several hours to generate an image.
The T-shaped probe also leads to superior spatial resolution. The best images from a conventional tip have tens of nanometres of spatial resolution in liquid, and cannot visualizing single molecules — whereas Sahin’s tool has a typical resolution of one nanometre.
Easy to manufacture
Sahin also says that the cantilevers are “relatively easy to manufacture,” — the team’s probes are made by an AFM cantilever manufacturer that carries out bespoke batch production. The experiments were done on a commercial AFM.
A protein’s mechanical properties can also be determined by inelastic neutron scattering (INS). However, neutron sources are only available in national facilities, and protein replicas with certain hydrogen atoms replaced with deuterium are needed.
Despite these drawbacks, INS has been used to study bacteriorhodopsin. “There is an agreement in the numbers, but that does not necessarily build additional confidence into our method,” says Sahin. INS quantifies thermally actuated atomic fluctuations, while Sahin’s team has determined the collective response of atoms to external forces, and he says that the correlation between these two techniques is unknown.
Future targets
The next goal for Sahin’s team is to measure protein dynamics. “High-speed measurements can, in principle, show changes in protein that occur at the microsecond to millisecond timetable.” Tracking of single molecule dynamics might even be possible, because enzymes form and break chemical bonds on this timescale.
The research is published in the journal Nature Nanotechnology.