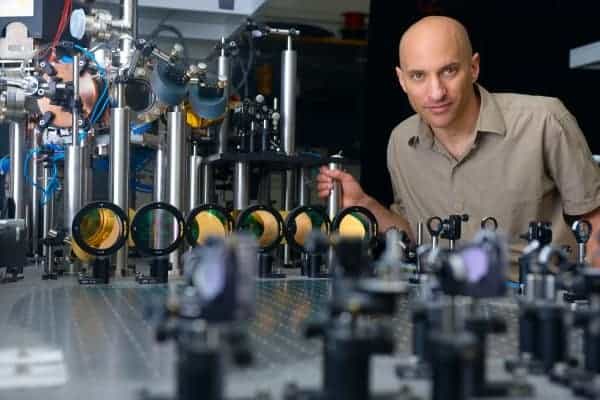
Physicists in Israel have created a black–hole analogue that can trap sound in the same way an astrophysical black hole can trap light. The system, which comprises a “density-inverted Bose–Einstein condensate”, may present one of the best chances yet to detect elusive Hawking radiation.
In an astrophysical sense, a black hole is a region of space so dense that the gravity at its centre approaches infinity. Surrounding this region is the so–called event horizon, beyond which nothing — not even light — can escape.
For a long time, the notion that black holes are totally black led scientists to think the objects could not be observed directly. But in the early 1970s, Stephen Hawking, building on work by Jacob Bekenstein of the Hebrew University of Jerusalem, showed that this need not be the case. Hawking’s calculations indicated that if a particle–antiparticle pair came into existence straddling the event horizon, the one closest to the black hole would fall inwards while the other would escape. The sum of escaped particles would constitute Hawking radiation, and could reveal the black hole’s presence.
The trouble is that the temperature of Hawking radiation would be much lower than the universe’s background radiation, and therefore would be difficult to make out. For this reason, several research groups have attempted to create analogues of black holes in the lab, where they can decrease the background temperature. However, so far none of these systems — which include optical fibres and quantum fluids — have yielded Hawking radiation that could be detected.
Two potentials
Jeff Steinhauer and colleagues of the Israel Institute of Technology “Technion” in Haifa have perhaps got a step further with their sonic black hole. Their system comprises a Bose–Einstein condensate (BEC), or a collection of cold atoms that move coherently in the same quantum state.
The BEC contains two potentials: one crater-shaped “harmonic” potential, which is formed via magnetic fields, and a deeper, superimposed “Gaussian” potential, which is formed with a laser beam. By shifting the potential sideways, the researchers found that atoms fell in and climbed out of the potential at a speed faster than sound in the medium, or about 1 mm/s.
This supersonic travel is key to the success of the system. If a sound wave approaches the atoms in the opposite direction as their movement, it will reach the atoms but will never be able to leave. In this instance, the moving atoms act as a sonic “black hole”, from which no sound can leave. Conversely, if the atomic flow “velocity gradient” is altered, the sound wave will never reach the atoms, in which case they act as a sonic “white hole”..
The Israeli group’s evidence for this effect came by mapping the density of the atomic clouds in and around the Gaussian potential minimum. Although they do not yet have evidence for Hawking radiation — which in this type of system would constitute packets of sound energy or “phonons” — they predict the Hawking temperature to be at a temperature in the region of 0.3 nK. Just an order-of-magnitude increase in this temperature should be enough to make the Hawking radiation visible, they say.
Good system
Renaud Parentani, a theorist at the University Paris-Sud in France who studies black-hole analogues, thinks the Israeli group’s experiment marks “a new step” towards seeing Hawking radiation in a condensed–matter environment. In particular, he has done calculations to show that this type of quantum–fluid system should suffer less from dispersion — which limits the production of particle–antiparticle pairs at the event horizon — than other recently tried systems, such as fibre optics.
Parentani says that if the researchers can achieve an order-of-magnitude increase in temperature, it could become easier to detect Hawking radiation. However, he points out — as do the researchers themselves — that they may still see it without such an increase if the black– and white–hole analogues work together to amplify the signal. In this theory, which was devised by Steven Corely of the University of Alberta, Canada, and Ted Jacobson of the University of Maryland, US, in 1999, the system behaves like a laser, so that the Hawking radiation becomes more intense than the temperature alone would imply.
The research is described in detail at arXiv:0905.0777.