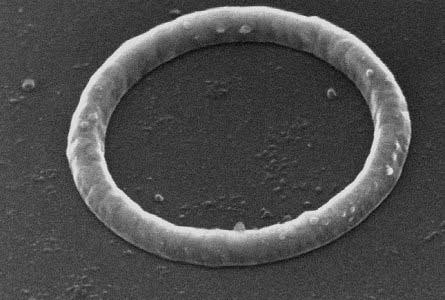
If you want an electrical current to flow around a normal metal ring you have to supply enough energy to overcome the metal’s resistance – right? Not always, according to physicists in the US and Germany who have the best experimental evidence yet that currents can flow forever in micrometre-sized metal rings. The research involves measuring the tiny magnetic fields associated with the currents and validates a theory of persistent currents that was proposed in 1969.
Physicists are familiar with persistent currents in superconductors – in which electrons can flow forever, unhindered by resistance. But even the best normal conductors such as copper or gold have electrical resistance due to electrons scattering from defects, which should make persistent currents impossible.
However, if a metal ring is very small – about 1 μm diameter or less – quantum mechanics says that its electrons should behave in much the same way as electrons orbiting an atomic nucleus. And in the same way that electrons in the lowest energy configuration of an atom maintain their orbits without the constant input of energy, electrons in such “mesoscopic” rings should flow forever – even if the ring has resistance due to defect scattering.
Indeed, a 1 μm diameter ring cooled to 1 K should support a current of about 1 nA.
Breaking the ring
Nanoampere currents can be measured with an ammeter, but this would involve breaking the ring to include the ammeter in the circuit. Even if the ammeter could be shrunk down to micrometre size, its presence would destroy the quantum coherence that allows the current to flow.
Instead physicists have used superconducting quantum interference devices (SQUIDs) to try to measure the tiny magnetic fields created by persistent currents. This is very difficult because SQUIDs are sensitive to magnetic impurities in the rings. In addition, a magnetic field must be applied along the ring’s axis to cause the persistent current to flow in one direction. Such a field makes it difficult to operate a SQUID – but without an applied field some electrons would flow clockwise and others anti-clockwise, resulting in zero net current.
Because of these problems, experimental results have been inconsistent and at odds with theoretical predictions. Now, Jack Harris and team at Yale University along with a colleague at the Free University of Berlin have invented a completely new way of measuring persistent currents that is about 100 times more sensitive than SQUID-based experiments.
Tiny diving boards
The team grew aluminium rings on a silicon chip and then used lithographic processes to create 300 nm-thick diving-board-like cantilevers with one or more rings at the tips.
To measure the current in the rings a cantilever is aligned at an angle of about 45° to a strong magnetic field of several Tesla. The component of the applied magnetic field perpendicular to the cantilever causes the persistent current to flow in one direction – resulting in an additional magnetic field perpendicular to the cantilever. The parallel component of the applied field is at right angles to the field created by the persistent current, resulting in a torque on the cantilever.
The cantilever has a natural frequency of oscillation, which changes as a result of this torque. By comparing the frequencies with and without the applied field, Harris and colleagues can work out the size of the persistent currents in the rings.
The team studied several different cantilevers decorated with a single ring or arrays of hundreds or thousands of identical rings. The rings on different cantilevers had diameters varying from 616 nm to 1.59 μm.
Closing a chapter
By measuring the size of the persistent current while changing the magnetic field, the team confirmed that the persistent current is a periodic function of the magnetic flux quantum h/e – as predicted by Yoseph Imry of Israel’s Weizmann Institute and colleagues in 1969 and 1983. Imry described the study as a “very thorough study” that has “closed a chapter on persistent currents”.
He pointed out, however, that Harris and colleagues did not study persistent currents at low magnetic fields, where the currents are expected to be a periodic function of h/2e. “The explanation is akin to the simpler one involving ‘coherent backscattering’, the addition of paths encircling the ring in opposite, time reversed, orbits”, explained Imry. Imry told physicsworld.com that Harris should be able to do experiments at lower field strengths in order to see the h/2e regime.
Harris intends to do further experiments at different magnetic field strengths and angles, as well as different temperatures and ring size. He also said that he is keen to put tiny structures into the rings such as quantum dots or Josephson junctions – and study the resulting mesoscopic circuits remotely using the cantilever technique.
The work is described in Science.