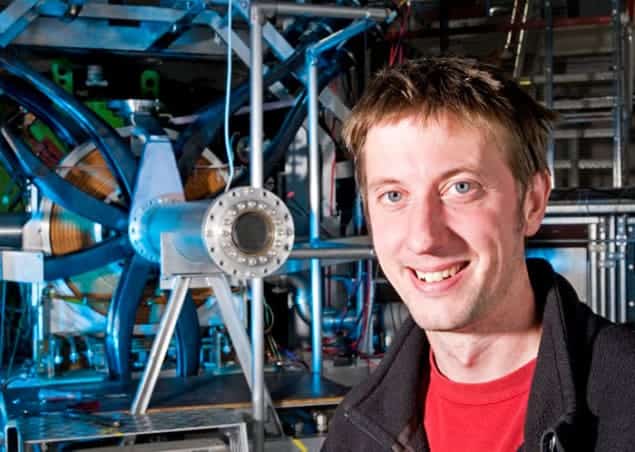
Researchers in the UK and France have measured the charge and current of “magnetic monopoles”, which were recently shown to exist in materials called spin ices. While the team didn’t actually create the magnetic analogue of an electrical circuit, they showed that magnetic monopoles respond to magnetic fields in much the same way as charged particles respond to electric fields.
Earlier this year two independent groups of physicists provided the best evidence yet that magnetic monopoles – free “north” and “south” magnetic poles – can exist in magnetic materials called spin ices.
The magnetic moments in a spin ice do not line up like those in a ferromagnet. Instead physicists believe that they join up to create magnetic flux lines within the material that resemble a knotted mess of strings. However, if a moment is flipped – a string is broken and the magnetic flux spills out in a manner resembling a monopole.
In September, teams led by Jonathan Morris at the Helmholtz Centre in Berlin and Tom Fennell at the Institute Laue-Langevin (ILL) in Grenoble measured the thermodynamic and other properties of two different spin ices – and their results suggested the existence of monopoles.
Charge and current
Now Fennell has joined forces with Steve Bramwell of University College London and Sean Giblin of Rutherford Appleton Laboratory (RAL) to make the first measurements of the magnetic charge and current associated with magnetic monopoles in the spin ice Dy2Ti2O7.
They did this by “mapping” the problem onto Lars Onsager’s 1934 theory of electrolytes – which describes how an applied electric field causes molecules in solution to ionize, thus boosting the conductivity of the solution. Working at RAL’s ISIS muon facility, the team applied a magnetic field to the spin ice, which creates north and south monopoles that drift apart to create magnetic currents in the material.
The presence of these currents was detected using muon spin resonance (µSR) – whereby a beam of muons is fired at the material and some of the particles take up residence in the crystal lattice. The muons decay to produce positrons, which exit the material and are detected. The direction of the emitted positron is related to the magnetic polarization of the muon.
Wobbling muons
In the absence of monopoles, a muon’s magnetic moment will wobble about an applied magnetic field, and the µSR signal resembles a decaying sine wave. The creation of monopoles leads to random, local magnetic fields, which disrupt the rotation and hasten the decay of the µSR signal.
The team related the µSR decay rate to the magnetic conductivity of the spin ice – which increased with stronger applied magnetic fields, just as predicted by Onsager’s theory. This allowed the team to use the theory to determine the elementary magnetic charge of the monopoles – which was in good agreement with the theoretical value.
Claudio Castelnovo of Oxford University, who was not involved in the research, described the work as “an important paradigm shift in spin ice research” and “the first step in generating a current of monopoles”.
Explaining the inexplicable
Fennell told physicsworld.com that the study could also explain the “inexplicable behaviour” that has been seen in previous µSR studies of spin ices. “The experiments could have been seeing monopoles all along,” he said.
The spin-ice monopoles have very different origins from the monopoles famously predicted by Paul Dirac’s work on quantum electrodynamics. But, because the monopoles occur in magnetic materials, understanding their properties could help with the development of magnetic memories and other spintronic devices.
The results are published in Nature.