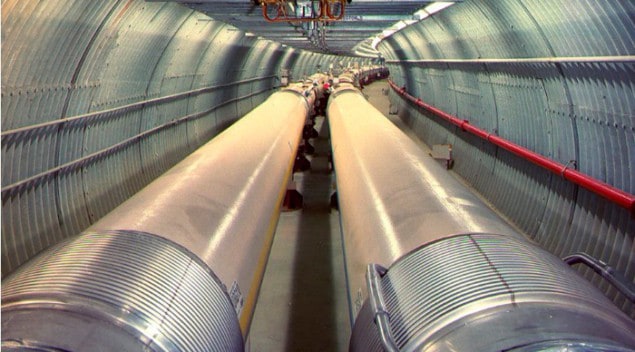
Light pulses emitted by an exotic state of matter known as a quark–gluon plasma last for just a few yoctoseconds – according to calculations by physicists in Germany. One yoctosecond is one trillionth of a trillionth of a second (10–24 s) and is comparable to the time it takes light to cross an atomic nucleus. Indeed, the researchers say that such pulses could be used to study the ultrafast processes taking place inside nuclei.
Standard ultrafast lasers can produce pulses no shorter than a few femtoseconds (10–15 s) long. It is, however, possible to generate attosecond (10–18 s) pulses by combining the frequency harmonics that result from the nonlinear interaction of femtosecond pulses with various atoms.
Now, Jörg Evers and colleagues at the Max Planck Institute for Nuclear Physics in Heidelberg have worked out that it should be possible to extend this lower limit down by a further factor of a million.
Free soup
Their approach uses the light emitted by a quark–gluon plasma, a soup of free quarks and gluons, the force carriers that normally bind quarks together inside protons and neutrons. This state of matter is believed not to have existed naturally since the universe was just a millionth of a second old but can be recreated by smashing heavy ions into one another at extremely high energies inside particle accelerators. It is currently produced by the Relativistic Heavy Ion Collider (RHIC) at the Brookhaven Laboratory in New York and will also be created inside the Large Hadron Collider, due to switch on shortly at CERN in Geneva.
Evers and co-workers argue that a quark–gluon plasma naturally generates extremely brief photon pulses as it cools down. The plasma is initially very hot but then cools rapidly as it expands to about the size of a nucleus, at which point it turns back into normal matter. High-energy photons can only be emitted by the hot plasma itself and not the resulting normal matter, which means that pulses of such high-energy photons cannot last for longer than the lifetime of the plasma itself – which is just a few yoctoseconds. The trick is to simply focus on these photons, rather than those with a lower energy.
Two pulses are better than one
Establishing whether or not a quark–gluon plasma produces yoctosecond pulses is, however, not enough to show that it could be used to actually measure such brief intervals of time. This requires a double pulse: one to prepare the system and the other to carry out the measurement. Evers illustrates this with reference to a sprint race, in which the sound of a starter pistol sets the runners off and a photograph taken a few seconds later then establishes their position after that time.
Fortunately, a quark–gluon plasma could produce such a double pulse as it expands. Evers explains that initially the plasma emits light in all directions but then a competition of two mechanisms sets in. The rapid expansion of the plasma along the original collision axis of the heavy ions dictates that after some time most of the remaining particles within the plasma should move at right angles to this axis, which also constrains the light to be emitted in this direction. But a few moments later the complex internal dynamics of the plasma renders the motion, and with it the light emission, isotropic again. This should mean that a detector placed close to the collision axis will register a signal at the beginning and end of the plasma’s expansion, but not in-between: this is the double pulse.
According to Evers, this technique could shed light on the very dynamics inside a quark–gluon plasma that generates the double pulse. He says it could also be used to study processes inside atomic nuclei, perhaps improving our understanding of the reactions inside compact stars or supernovae, or helping us to design better fusion reactors. Indeed, he notes that measurements on the femtosecond timescale have already been used in industrial applications, such as investigating combustion processes when designing new engines.
Measurement technology needed
John Tisch, a laser physicist at Imperial College London questions how easy it will be to build the technology to measure yoctosecond pulses but adds that people had similar doubts about attosecond technology. “The key to unlock attosecond science was to utilize aspects of the generation process itself to measure the pulses,” he said. “So I would anticipate that yoctosecond pulses – if they ever materialize – might be measured by turning some of the same physics governing their generation back on itself.”
Evers says that he and his colleagues are currently developing yoctosecond detector technology but are not giving any secrets away.
The research is published in Physical Review Letters.