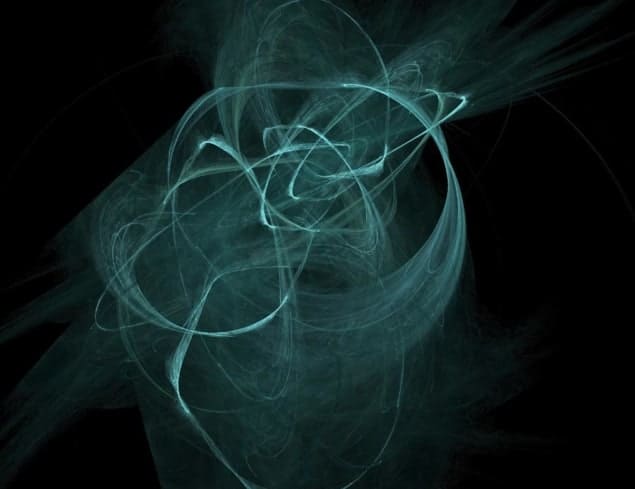
Fractal patterns are ubiquitous in nature from the shape of a galaxy to the structure of a snowflake. They may also lie behind the mysterious phenomenon of high-temperature superconductivity, according to a group of physicists in Europe who have observed the characteristic scale-invariant patterns in the structure of a superconducting copper oxide, using a new kind of X-ray microscopy.
High-temperature superconductivity was discovered in a class of ceramic compounds known as cuprates, which consist of layers of copper oxide sandwiched between other elements, by IBM researchers Georg Bednorz and Alex Müller in 1986. Since the discovery, scientists have identified cuprates that remain superconducting at temperatures as high as 135 K, but the mechanism behind the phenomenon remains a mystery. Unlike conventional superconductors, such as elemental mercury or lead, high-temperature superconductors do not appear to create the pairs of electrons needed for zero-resistance conductivity via vibrations of the crystal lattice.
Could be in the oxygen stripes
Some theoretical physicists have suggested that high-temperature superconductivity may be linked to the distribution of oxygen ions in the layers between the copper oxide. They say it might be the formation of some of these ions into rows, or “stripes”, that is responsible for cuprates’ remarkable conduction properties.
To investigate these claims, a team including Antonio Bianconi at the University of Rome “La Sapienza” exposed a sample of the superconductor lanthanum copper oxide to X-rays generated at the European Synchrotron Radiation Facility in France. By using advanced X-ray optics, the researchers were able to focus the powerful beam down to a spot one-millionth of a metre across and perform X-ray diffraction analysis over this tiny area. Scanning the beam across the sample and obtaining a scattering pattern for each square micron of the sample, Bianconi and colleagues obtained an extremely detailed picture of the superconductor’s structure, with high scattering intensity corresponding to greater structural order.
What they found was that this intensity followed a power-law distribution, in other words that the superconductor was made up of a small number of very high-ordered regions and larger numbers of disordered regions. This, they say, is the hallmark of a scale-free distribution, which is typical of a fractal pattern – with the oxygen stripes forming a similar structure on all scales up to 400 µm.
Fractal scales with temperature
In addition, the researchers found that this fractal distribution increases the temperature up to which the lanthanum copper oxide remains superconducting. They altered the transition temperature of the sample by heat treatment and then recorded its X-ray diffraction image. Carrying out this process at five different transition temperatures, they found that the higher this temperature the more closely the intensity pattern resembled a power law.
To try and explain this correlation, Bianconi suggests that the fractal distribution of oxygen ions makes the ordered and disordered regions of the superconductor very highly interconnected, which maximizes the interference between the wavefunctions of the superconducting condensates of these two regions. This, he says, increases the stability of the quantum coherence that is responsible for superconductivity, rendering the superconducting state robust at higher temperatures.
Nigel Hussey of the University of Bristol describes the research as a “beautiful example of self-organization and complexity in a transition metal oxide” but is not convinced that the current result sheds further light on the cause of high-temperature superconductivity. “Crystalline order does not cause superconductivity, but it can improve it,” he says. “Likewise, disorder can weaken it.”
This research is described in Nature.