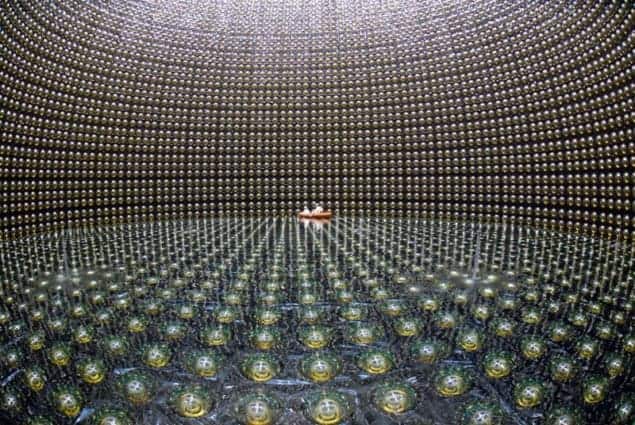
Physicists in the US and Canada have proposed a new particle that could solve two important mysteries of modern physics: what is dark matter and why is there much more matter than antimatter in the universe?
The yet-to-be-discovered “X” particle is expected to decay mostly to normal matter, whereas its antiparticle is expected decay mostly to “hidden” antimatter. The team claims that its existence in the early universe could explain why there is more matter than antimatter in the universe – and that dark matter is in fact hidden antimatter.
Dark matter is a mysterious substance that appears to make up about 80% of the material universe. Although its existence can be inferred from its gravitational pull on normal matter, physicists have yet to detect it directly and therefore don’t know what it is made of. Antimatter, on the other hand, is easy to create and study in the lab. However, the Standard Model of particle physics cannot explain why antimatter is so rare in a universe that is dominated by matter – a mystery called baryon asymmetry.
Hypothetical and hidden
Now, Hooman Davoudiasl of Brookhaven National Laboratory and colleagues at TRIUMF and the University of British Columbia have proposed a new particle dubbed X that could solve both of these mysteries. X has a mass of about 1000 GeV – making it about a thousand times heavier than a proton. This particle can decay to a neutron or to two hypothetical hidden particles called Y and Φ. Both hidden particles would have masses of about 2–3 GeV. Its antiparticle, anti-X, decays to an antineutron or to the pair anti-Y and anti-Φ.
Physicists have tried to try to explain the baryon asymmetry by invoking a violation of the charge–parity (CP) symmetry – the result being that decaying particles are more likely to generate matter than antimatter. CP violation has been observed in laboratories, but the preference for matter is far too small to account for the proportion of matter in the universe.
X also commits CP violation in a way that author Kris Sigurdson of the University of British Columbia calls a “yin yang” decay pattern. While X decays to neutrons more often than anti-X decays to antineutrons, it is balanced by anti-X, which decays to anti-Y and anti-Φ more often than Y and Φ. When almost all particles with an available antiparticle annihilated one another in the early universe, these discrepancies left a chunk of visible matter and a heavier chunk of dark antimatter to form the cosmos.
Look for proton decays
The team has also thought of how the anti-Y and anti-Φ particles could be detected. Unlike weakly interacting massive particles (WIMPs) – which dominate many theories of dark matter – anti-Y and anti-Φ do not annihilate each other. However, the antiparticles would cause protons to decay, which is forbidden by the Standard Model. If an anti-Y particle collides with a proton, for instance, a virtual interaction with particle X can break the proton apart, transforming it into a positively charged kaon, and turning the anti-Y particle into a Φ particle.
A detector looking for proton decays, such as SuperKamiokande in Japan’s Kamioka mine, could catch the kaon. Kaons produced this way would have much higher energies than those generated by proton decays allowed by other theories that go beyond the Standard Model. Although protons are expected to be fairly resilient to this decay process, Sigurdson says, “This scenario could be on the boundary of detectability.”
“It looks like a very interesting model,” says Dan Hooper of Fermilab. Although at least three more models linking the production of dark matter to the baryon asymmetry are in development, he says that the proton-decay signature sets this scenario apart.
Driven by experiments
Matthew Buckley of Fermilab says that there is a sudden interest in linking dark matter with the baryon asymmetry because of recent experiments that have tried (unsuccessfully) to detect dark matter. Although WIMP models prefer dark-matter particles with masses around 100 GeV, the experiments suggest that dark-matter particles have masses nearer 7 or 8 GeV.
Having such a small mass “definitely isn’t what a WIMP is ‘supposed to look like’,” says Buckley. However, dark matter that also explains the baryon asymmetry seems to be more in line with recent experimental results – which is why Buckley believes it deserves further exploration.
The work is reported in Phys. Rev. Lett. 105 211304.