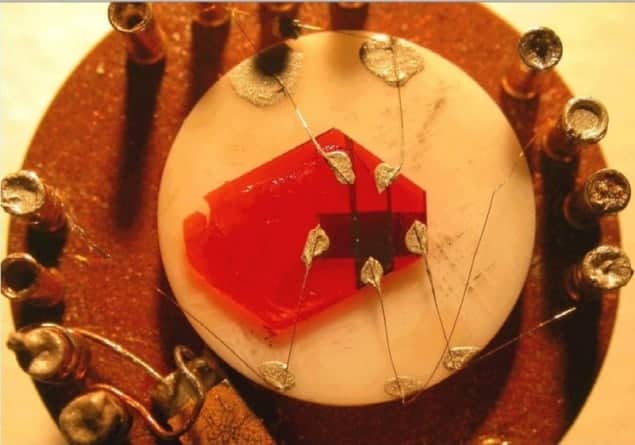
Physicists in the US have shown that organic semiconductors may be just as promising as their inorganic counterparts in solar cells. The researchers’ discovery – that bound electron–hole pairs can travel a thousand times farther in organic semiconductors than previously observed – suggests that organic solar cells could one day be made efficient, cheap and in high volume.
Today, commercial solar cells are made of inorganic semiconductors such as silicon. When a photon in the visible or near-infrared part of the light spectrum strikes the surface of the cell, it generates an electron–hole pair, which quickly disassociates. It is effectively the separation of such electrons and holes in the semiconductor that creates a voltage, so that a current can flow.
But inorganic solar cells are expensive to produce, and for this reason researchers have long considered organic alternatives. Organic semiconductors can often be made inexpensively in solution, and indeed are already used commercially to make light-emitting diodes (LEDs) and flat-screen displays. They also tend to have a lower impact on the environment than their inorganic counterparts.
Holes in the plan
The problem with organic semiconductors, however, is the separation of electrons and holes. When an electron and a hole are created, they are bound strongly into a quasi-particle known as an exciton, and the only way they can separate is to reach an artificial “heterojunction” and shift either side. Unfortunately, in the organic semiconductors tested in the past, excitons have only been able to travel a few nanometres, which means the cells must be made very thin and, ultimately, inefficient.
Vitaly Podzorov and colleagues at Rutgers University, New Jersey, have now discovered an organic material in which excitons can travel roughly a thousand times farther, of the order of several micrometres. “The further excitons can migrate in a material, the better our chances of collecting many of them at heterojunctions, where they contribute to the generation of electricity by splitting into free electrons and holes,” says Podzorov.
The material in question is rubrene, a highly ordered organic semiconductor that is already used in organic LEDs. Excitons are electrically neutral and are therefore difficult to measure in the bulk, so the Rutgers researchers developed their own method whereby they measured the current of disassociated excitons leaving the material’s underside. The team was able to build a clear picture of the exitons moving through the material by probing it with a light source, where varying the wavelength and polarization enabled the penetration depth of the light to be altered.
Scaling up
Bernard Kippelen, an electrical engineer at the Georgia Institute of Technology in the US, points out a couple of drawbacks with rubrene as an organic semiconductor. He believes it does not absorb as much of the light spectrum as many inorganic semiconductors, and researchers do not yet have a means of fabricating it on large scales. Nonetheless, he says it does highlight the potential for organic solar cells.
“For a long time, researchers in the community thought excitons could not really diffuse more than 10 nanometres, so that the only way forward was to make heterojunctions in the bulk,” he explains. “This work shows that the exciton-diffusion bottleneck, as people refer to the problem, is not unsolvable.”
The Rutgers team believes the reason for rubrene’s superior performance lies in both its highly ordered structure and in the nature of the excitons generated. Rather than staying as single excitons, Podzorov and colleagues think the electron–hole pairs “split” into lower-energy triplet excitons, which have longer lifetimes and thus can travel farther.
According to David Beljonne, an expert in organic semiconductors at the University of Mons in Belgium, this also has its drawbacks. Since triplet excitons have a lower energy, they create a smaller voltage in a solar cell and therefore generate less power. “It clearly emphasizes that in the business of photovoltaics, it is difficult to optimize simultaneously all intervening electronic processes,” Beljonne says. “In other words, one might win here and lose there. But it does open up new avenues as triplets had previously been thought of as sinks for charge generation.”
This research is described in a paper in Nature Materials.