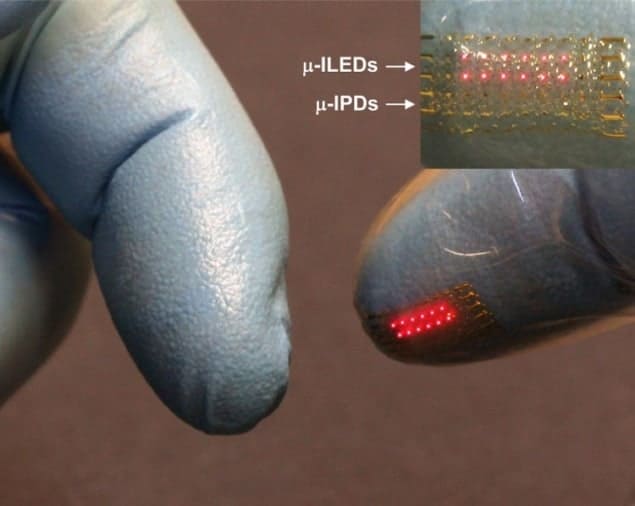
Light-emitting diodes – or LEDs – have already found their way into traffic lights, TV screens and incredibly efficient light bulbs. And it may not be long before they are also deployed in medical technologies such as proximity-sensing gloves, surgical threads and intravenous flow rate monitors, thanks to the efforts of an international team headed by John Rogers from the University of Illinois, Urbana Champaign.
To target these applications, Rogers and colleagues have exploited a new printing technique for forming arrays of incredibly small red LEDs on flexible substrates.
Like conventional LED manufacture, device fabrication begins by depositing a stack of compound semiconductor layers onto a substrate. The lower and upper layers have an excess and a deficiency of electrons, respectively, and sandwiched between them is a layer just a few nanometres thick, known as a quantum well. When a voltage is applied across the entire structure, electrons and their positive counterparts, known as holes, are driven into the well where they combine to emit light.
LEDs are normally made by sawing wafers into thousands and thousands of square-shaped chips. To prevent them from becoming too fragile, their edges are at least 300 µm – far too long for forming flexible arrays on plastic sheets.
Small enough for flexibility
To produce LEDs with sides of just 50 µm, Rogers and his co-workers define the chip area with photolithography and etching. A printing technique then transfers arrays of these LEDs to alternative substrates, where they are given electrical contacts and wired in series.
“Printing is a key part of the process,” says Rogers. “We have developed that technique to a very high level of sophistication, and we now have yields of over 99% and [placement] accuracies of about one micron.”
While light-emitting organic materials could have substantially simplified the process of making LEDs on a flexible substrate, organics have other shortcomings “Their brightness can’t compete with inorganic LEDs, and encapsulating them to prevent exposure to minute levels of moisture and oxygen is extremely difficult,” explains Rogers.
Stretching, flexing, twisting and bending
To test the robustness of its LED arrays, the team deformed them and monitored changes in performance. “We can accommodate nearly any type of deformation mode – even to extreme values of stretching, flexing, twisting and bending – up to 100,000 cycles or more.” Another strength of the printing process is that it can form LED arrays on a vast range of substrates, including plastic, rubber, aluminium foil, paper and even tree leaves.
To demonstrate potential uses for tiny LED arrays, the researchers attached them to a tube to provide a light source for a medical device that measures the concentration of glucose delivered intravenously.
Another application for these arrays is surgical stitching. It is not possible to print the devices onto a thread, but they can be attached by rolling this material over a glass carrier that is populated with red LEDs. Stitches incorporating these tiny light emitters have been inserted into an anaesthetized mouse. According to the team, adding LEDs to surgical thread delivers multiple benefits: accelerated healing; illumination of deep tissue; and the opportunity to monitor blood oxygenation.
Light at your fingertips
LED arrays can also be deployed in the fingertips of gloves to create proximity sensors for aiding robotic systems or surgical procedures. To do this, the researchers have integrated tiny LEDs with similar-sized photodetectors. This allows the distance to the nearby object to be determined through measurements of the intensity of backscattered light.
Rogers says that a start-up company, MC10, is targeting commercialization of some of the team’s technology
“From the science and materials side, we are working to implement related ideas with blue and ultraviolet LEDs, to expand the functionality.”
The researchers report this work in the latest edition of Nature Materials.