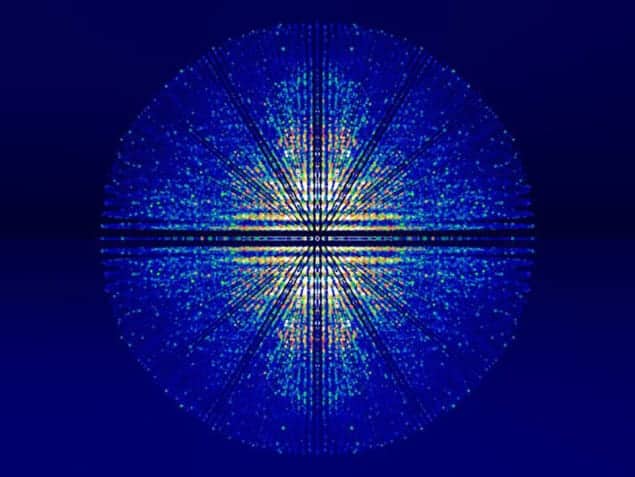
A new X-ray technique with the potential to image biological samples of any size has been unveiled by an international team of scientists. The breakthrough involves flashing a sample with intense X-rays before any radiation damage sets in and should allow researchers to analyse the structures of proteins and other samples that have never been imaged before.
X-rays are one of the most important tools to study the structures of biological samples. Typically a sample must be crystallized so that the molecules line up into a regular arrangement. When the X-rays pass through the crystal they diffract, producing a distinctive pattern from which scientists can deduce the sample’s structure.
But crystallizing biological samples isn’t easy and it is often hard to end up with crystals more than a hundred or so microns in diameter. If the crystals are too small, researchers have to expose them with more X-rays to get a good diffraction pattern – and this risks destroying the samples altogether.
The pulse literally outruns the damage Henry Chapman, DESY
Now, in two papers published in Nature, multidisciplinary teams of researchers from Sweden, Germany, France and the US have demonstrated a way to circumvent this problem. Using the Linac Coherent Light Source (LCLS), an X-ray free-electron laser based in California, they expose tiny biological samples – both crystalline and non-crystalline – to flashes of X-rays rather than continuous beams. “We get around the problem of radiation damage by using pulses so short and so intense that the light pulse ‘flash’ is over before any effect of the damage manifests itself,” says Henry Chapman, lead author of one of the studies, who is based at DESY in Hamburg, Germany. “The pulse literally outruns the damage.”
Notoriously difficult molecule
Chapman’s group used the X-ray flashes from the LCLS to image tiny crystals of a membrane protein molecule that is notoriously difficult to grow into crystals large enough for conventional X-ray imaging. The molecules are suspended in water jets and exposed to X-rays to generate a diffraction pattern with a resolution of 0.85 nm. The pattern is proof that the crystals remained intact during the exposure. By inputting the diffraction measurements into a computer program, the researchers were able to view the crystal structure.
Meanwhile, a group led by Marvin Seibert, Tomas Ekeberg and Filipe Maia of Uppsala University, Sweden, used the same technique to image a non-crystalline single particle – a “mimivirus”, the largest known virus at 450 nm in diameter. The lack of a crystalline arrangement here produced a continuous diffraction pattern, which had to be processed using a more advanced computer algorithm. The ultimate resolution of the structure was 32 nm.
“The technique is revolutionary, but it’s not yet of course at its limits,” says Christian Riekel, an expert in biological imaging at the European Synchrotron Radiation Facility in Grenoble, France. Riekel, who was not involved in either project, suggests that technological improvements, such as reducing the wavelength, the focus size, the flash length and the number of samples in the water jet will improve clarity. “What you see is more of a proof of principle,” he adds.
Crystals not needed anymore?
Elspeth Garman, a molecular biophysicist at the University of Oxford, UK, has praise for both experiments. For the study of non-crystalline samples, Garman says it remains to be seen whether smaller particles, such as living cells, can be imaged. “If higher resolution can indeed be achieved, the great advantage that this technique will have over existing structural determination methods of large proteins and their complexes is that crystals will not be necessary at all,” she says.
“I must admit to having been sceptical in the past about the possibility of this developing into a useful technique, mainly because of the radiation damage issue, but also because of the limited resolution,” she adds. “However, following this report, I am very much looking forward to being proved wrong.”
Chapman believes there might be even more to come with the technique. He says that it should be possible to take multiple shots of a sample, to create an X-ray “movie”. “The X-ray pulses are so short that you should be able to watch the chemistry in action,” he adds.
The work is reported in Nature 470 78 and Nature 470 73.