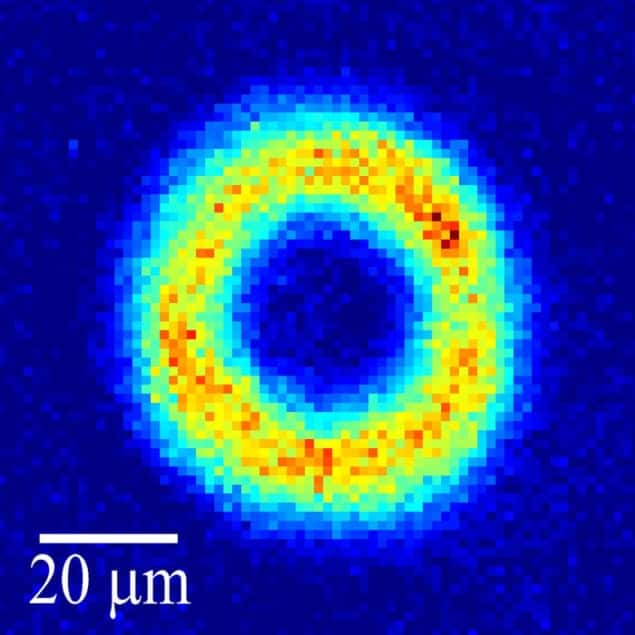
The first circuit of ultracold atoms has been unveiled by physicists in the US. The team has already shown how the atomic current in the circuit can be controlled precisely and believes that similar circuits could be used in ultra-sensitive rotation sensors.
Previously, physicists have created circuits of superfluid helium – a zero-viscosity liquid state that occurs at temperatures below about 2 K. However, circuits of much more dilute ultracold atoms are more interesting to physicists because the interactions between atoms are weaker and are easier to describe mathematically. As a result, ensembles of ultracold atoms are routinely used to study the physics of quantum systems.
Kevin Wright and colleagues at the Joint Quantum Institute in Gaithersburg, Maryland created their circuit from a Bose–Einstein condensate (BEC) of sodium atoms. BECs are formed when identical atoms with integer spin are cooled until all the atoms are in the same quantum state – and behave as a single quantum particle.
The team began by laser cooling sodium atoms in a beam and capturing about a billion of them in a magnetic trap. The strength of the trapping field is then reduced to allow the more energetic atoms to bounce out, further reducing the temperature of the remaining atoms. The result is a collection of about 300,000 atoms at a temperature of 10 nK, which is cold enough to form a BEC.
Flattened doughnut
Then, the team caught the BEC in crossed lasers, dropping the magnetic trap. One laser beam was cylindrical while the other was sheet-like. Together, these two lasers set up a field that causes the sodium atoms to collect in a space shaped like a flattened doughnut, with a radius of about 20 µm.
According to Wright, the smoothness of the optical container created by the two lasers is crucial to the group’s success. “You can imagine that if you have a bucket that’s really bumpy, you just end up with a bunch of little pools,” he explained. But in the carefully crafted laser field, the atoms were able to settle evenly in the ring, creating a continuous loop.
Also important is how the team starts the rotation without breaking up the BEC, which is done by shining two lasers at the condensate. The photons from one laser spiral, carrying angular momentum, but those from the other laser carry none. The lasers are tuned to force the atoms to absorb photons from one beam and emit them into the other. As a result, they pick up angular momentum, and the condensate begins to rotate.
Turbulent flow
The researchers were also able to boost the current by obstructing the spinning condensate. This is done by focusing blue laser light in the ring. The laser beam’s elliptical shape is broadside to the atom current, creating a shallow spot in the stream of sodium atoms. Like a river running through a shallow region, the current speeds up as it passes the obstruction. The taller the obstruction, the faster the current had to run over it, and the flow became turbulent at a certain critical speed.
This critical speed has caught the attention of other physicists, including Francesco Piazza of the University of Trento in Italy. “The question of superfluid critical velocity in the presence of constrictions is a long standing problem,” said Piazza. Their results seem to agree with the vortex picture put forward by Richard Feynman in 1955 and Piazza finds this interesting, although more accurate models are necessary for conclusive results.
The BEC is governed by quantum mechanics and therefore can only rotate at a set of discrete angular velocities. The researchers chose the slowest possible – about one circuit per second. Wright says the rotation itself can be imagined as a vortex trapped in the centre of the ring that can’t escape through the superfluid. But once the flow becomes turbulent, the vortex can get free of the loop, and so rotation halts.
As with many ultracold atom experiments, it is not possible to study the trapped BEC directly – instead it must be released from the trap in order to catch a glimpse of it properties. A flash from a laser casts a shadow of the atom cloud, revealing its density distribution. The size of the hole in the pattern is related to how fast the condensate had been rotating. The atoms tend to spread outward more than inward if they had been revolving quickly, but the hole disappears if the BEC had stopped spinning. The critical speed at which the rotation ceases in the condensate provides a benchmark for measuring rotation outside the device, which means that it could be used as a highly sensitive rotation detector.
Brian Anderson of the University of Arizona in Tucson believes that atom circuits could also for the basis of more complicated devices. “Understanding the response of an atomic superfluid to a barrier is therefore one of the first important steps towards the eventual realisation of such circuits,” he says.
A paper describing the work has been accepted for publication in Physical Review Letters and a preprint is available on arXiv – an analogue to the magnetic field-detecting superconducting quantum interference device (SQUID).