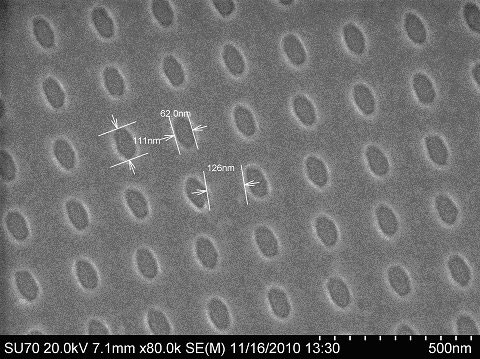
Tiny layered magnets could be used as the basic processing units in highly energy-efficient computers. So say researchers in the US who have shown that the magnetization of these nanometre-sized magnets can be switched using extremely small voltages that induce mechanical strain in a layer of the material. The resulting mechanical deformations affect the behaviour of electron spins, allowing the materials to be used in spintronics devices. These are electronic circuits that exploit the spin of the electron as well as its charge.
Hybrid spintronics/straintronics processors made from such magnets would require very little energy and therefore could work battery-free by harvesting energy from their environment. As a result they could find a host of unique applications, including implantable medical devices and autonomous sensors.
Modern-day computers store data on magnetic hard disk drives, in which the direction – “up” or “down” – of the magnetization in a small region of the disk corresponds to a binary bit (“1” or “0”, for example). Data are read by a magnetoresistance element and written by heating the bit with a laser and then flipping the moments with a magnetic field pulse from a tiny nearby coil.
Flipping bits with spin
More recently, researchers have experimented with the idea of exploiting “spin transfer torque”, whereby a current of spin-polarized electrons can flip the bits without the need for an external magnetic field. This idea can be taken further by using a current to move domain walls in a magnet and thus flip the bits in this way. However, the problem with all these approaches is that a relatively large amount of energy is needed for each bit flip – from fractions of a femtojoule to up to several femtojoules. Conventional transistor-based circuits dissipate similar amounts of energy per bit flip, giving no energy advantage to spin transfer torque devices.
Indeed, such a high energy requirement is a major obstacle to making ever smaller electronic devices that continue to obey Moore’s law – that the density of transistors integrated circuits doubles about every two years. So far, the silicon industry has kept the pace and transistors have exponentially decreased in size since down to about 20 nm today.
Now, Kuntal Roy and colleagues at the Virginia Commonwealth University claim that they can switch the magnetization of their nanomagnet-based devices with less than 0.4 attojoules of energy, a figure that is four orders of magnitude smaller than that for conventional transistors. “The energy requirement is so low that processors built with this technology could run by harvesting energy from their environment without needing a battery,” Roy told physicsworld.com.
Absorbing mechanical energy
The devices would do this by simply absorbing mechanical energy (in the form of vibrations) from their surroundings. This mechanical energy would be converted into electrical energy by electromechanical transducers that employ piezoelectrics, which are materials that generate tiny electric currents when stretched or compressed by vibrations.
Roy’s team designed a basic computer processing unit from a multiferroic nanomagnet consisting of a piezoelectric layer and a magnetostrictive layer in contact with each other. Magnetostrictive materials change shape when magnetized. When a voltage is applied to the piezoelectric layer, it generates a mechanical strain in the material. This strain is then transferred to the magnetostrictive layer, in which it causes stress, so flipping its magnetization.
Very small voltages
The amount of voltage needed to generate the necessary stress is extremely small, at around 10 mV, explained Roy. Since the energy consumed by the magnet is proportional to the square of the voltage, the energy required to switch a bit is also very small.
“Conventional transistors switch in 1 nanosecond by dissipating one million kT of energy in a circuit,” said Roy. “Our most recent results show that the multiferroic nanomagnets we used can switch in the same time while dissipating just 200 kT of energy in a circuit.”
Although previous research groups had also looked at encoding bits in magnetizations of nanomagnets, they did not use strain to switch the magnetization, but magnetic fields instead – something that requires a lot of energy. Indeed, the energy dissipated in these devices can be tens of millions of kT or more for switching in a nanosecond.
Monitoring brain waves
Potential applications for the new technology include medical devices, such as processors implanted in a patient’s brain that monitor brain waves to warn of impending epileptic seizures, said the researchers. “These devices would solely be powered by the head movements of the patient,” explained Roy.
Other applications include buoy-mounted computers, for example, that could follow temperature changes in the sea to help in weather forecasting. They would work by harvesting energy from the movement of sea waves. Processors mounted on bridges and other buildings could monitor structural deterioration by detecting acoustic vibration frequencies and so identify potentially dangerous fractures. “These devices would harvest energy from vibrations in the building structures caused by the wind or even passing traffic.”
The Virginia team is now busy fabricating real devices in the lab and hopes to demonstrate a working prototype very soon. It has also designed multi-state logic gates (four-state NOR gates) based on crystalline multiferroic nanomagnets. These devices could be used for “associative memory”, a type of device frequently employed for pattern recognition. “We should be able to build pattern recognizers that operate at very low energy cost – and so help contribute to so-called “green electronics”, added Roy.
Mimicking the human brain
And that is not all. Happily, the nanomagnets subjected to stress switch abruptly and the Virginia team believes that this behaviour could be used to design artificial synapses, which are central processing elements in neuromorphic networks that function by mimicking how the human brain works. The researchers are collaborating with a group from the University of Virginia and the University of Michigan to turn this idea into reality.
Some of the work mentioned here has been published in Applied Physics Letters while the rest is currently under review. Publications from the group can currently be read for free on arXiv.