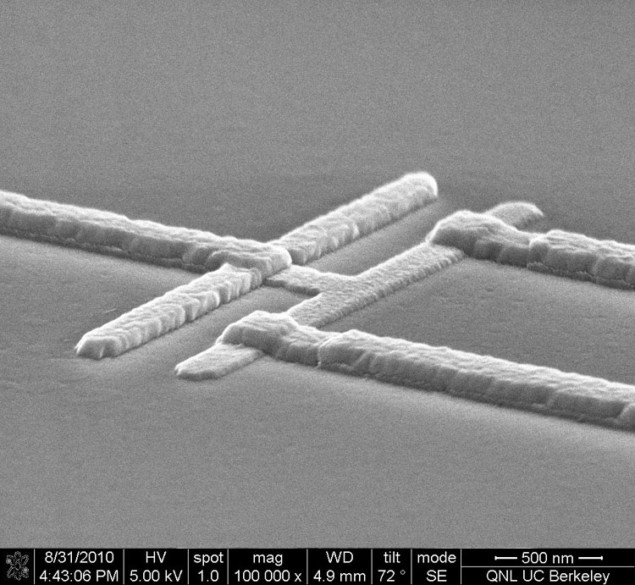
Researchers in the US say they are first to watch a macroscopic “artificial atom” jumping between energy levels in real time. The new capability to continuously monitor the energy states of a superconducting quantum bit, or qubit, could help to correct errors in quantum computations, tightening the race between these solid-state systems and quantum computers based on trapped atoms.
An optimal measurement system for quantum computations must meet three tough conditions. For one, it must rarely misidentify states. Second, the measurement can’t scramble the qubit’s state, which is tricky because quantum states are easy to destroy. And finally, it must be fast – on the timescale of nanoseconds. This is essential for seeing quantum jumps, since many measurements must be made before the qubit changes state.
While these conditions were met 25 years ago for trapped atoms, Rajamani Vijay, Daniel Slichter and Irfan Siddiqi at the University of California, Berkeley are the first to score the hat trick using superconducting qubits – sometimes referred to as artificial atoms because of their discrete energy states.
The team did the experiment inside a cryogenic helium refrigerator cooled to 30 mK. The superconducting qubit is an aluminium circuit, a few hundred microns across but considered macroscopic, and the low temperatures brought out its quantum properties. As a nonlinear electrical oscillator, its energy levels were unevenly spaced. This allows the team to use microwaves at a frequency of 4.753 GHz to drive it only between its ground and first excited states – the qubit’s 0 and 1 states.
Revealing and protecting
The researchers connect the qubit to the superconducting microwave cavity, an ordinary harmonic oscillator, through small capacitors. Because of this link, the cavity’s preferred photon frequency changes based on the state of the qubit. The cavity could reveal information about the qubit while at the same time protecting it from noise.
To measure the qubit’s state, the team generates higher-frequency microwave photons and admits them, no more than about 30 at a time, into the superconducting cavity. There, the photons interact with the qubit and acquire a phase shift depending on the qubit’s state – 180° if the qubit is in its excited state, or 0° if it is in the ground state.
Now bearing the qubit’s mark, the photons reflect out of the cavity toward the amplifier. Like the qubit, the amplifier is a nonlinear oscillator, this time designed to behave classically. Its superconductivity means low noise since it loses no energy as heat.
The amplifier is finely tuned to accept a particular power, or rate of incoming photons, without changing their phase as they reflect back out. This is precisely the power it receives from a microwave source, which generated frequencies matching that of the signal photons.
Minuscule but important
However, in joining this stream of photons on the way to the amplifier, the signal photons exert a minuscule but important influence – adding a tiny bit more power if their phases haven’t been shifted, or interfering destructively and slightly reducing the power if they have. The amplifier is so carefully balanced that it senses even this small difference and reacts dramatically. If the power is not what the amplifier expects, it imposes a phase shift of up to 90° in either direction on the photons it reflects. This shift is one way if the qubit is excited and the other way if it is in its ground state.
The amplifier magnifies the original signal from a few photons to hundreds of photons, making it large enough to withstand the noise introduced by common methods for increasing a signal. It also rapidly changes the phase of the photons, speeding up detection. “The combination of low noise and speed was crucial in observing quantum jumps for the first time,” says Vijay.
The team extracts the qubit’s state every 10 nanoseconds – plenty often enough to monitor the qubit’s 320 nanosecond long excited state and notice when it jumped to the ground state. And now that close surveillance on a qubit has been achieved, the method can be set to work correcting errors in quantum computations.
Correcting errors
To do this, a piece of quantum information is stored across multiple qubits. If one of these qubits falls out of its state, the others can still maintain the shared quantum information, as long as the wayward qubit is brought back into line quickly. But up until now, there was no way to continuously monitor a superconducting qubit and catch it making the transition from one state to another.
“Superconducting quantum bits are without doubt one of the hot candidates in the ongoing race towards a full-scale quantum computer,” says Jens Koch of Northwestern University in Evanston, Illinois. He calls the new monitoring system “a key step forward”.
A paper describing the work will be published next week in Physical Review Letters and a preprint is available on arXiv.