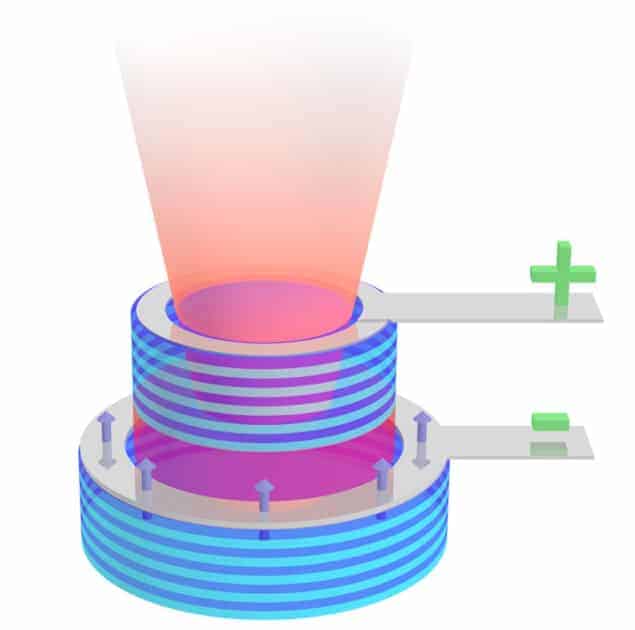
A new type of pulsed laser that modulates the polarization of its emitted light very rapidly has been created by researchers in Germany and Scotland. The polarization modulation occurs much faster than the intensity modulation normally used in optical telecoms systems – and the resulting polarization pulses could increase dramatically the speed of fibre-optic communications.
Modern telecoms systems encode information in pulses of laser light that are then sent along optical fibres. This is an incredibly efficient method of transmitting information, but its speed is ultimately limited by the rate at which the intensity of the laser can be modulated, since this dictates how long it takes to encode data into a train of pulses. With a traditional, intensity-modulated laser, the maximum achievable modulation rate is about 40 GHz.
Now Nils Gerhardt and colleagues at the Ruhr-Universitat Bochum, together with a colleague at the University of Strathclyde in Glasgow, have worked out a way to use electron spin to boost the modulation speed of a semiconductor laser – something that physicists have been working on for several years.
Lowering the energy threshold
In a standard semiconductor laser there are equal numbers of spin-up and spin-down electrons, so spin plays no part in its operation. However, physicists know that if the relative proportion of charge carriers in either spin state can be increased, this lowers the amount of energy that must be put into the laser before it starts emitting light – called the lasing threshold.
But sustaining this spin imbalance – or polarization – at room temperature has proven extremely difficult because thermal energy will randomize the spin in a few picoseconds. So Gerhardt and colleagues created waves of spin polarization in the semiconductor by blasting it with very short pulses of polarized light from another laser. While the electrons themselves still lose their spin polarization rapidly, some is passed on to photons, which then re-polarize the charge carriers. Such spin oscillations between photons and electrons last about 200 times longer than the electron spin polarization itself.
And there was a more interesting feature to the Bochum group’s laser. In contrast to the light from a standard semiconductor laser, which has no net polarization, the polarization of the light oscillated rapidly because of the coupling of the photon spins with the electron spins. While this switching had been demonstrated before, the polarization modulation rate had always been pegged to the intensity modulation rate.
To 100 GHz and beyond
Gerhardt and colleagues used their technique to modulate the polarization of the light from a 4 GHz laser at 11.6 GHz. This is still slower than state-of-the-art intensity-modulated lasers, but the researchers believe that it should be possible to improve on this. “Principally, you can go to over 100 GHz,” explained Gerhardt. “We’ve shown it theoretically, but first we have to develop a device and that’s what we’re currently doing.”
Physicist Igor Zutic of the State University of New York at Buffalo is impressed. “I would say this is a very exciting proof of principle and probably showing us the tip of the iceberg of what may be possible with these spin lasers,” he says.
However, Zutic and Gerhardt both agree that before such a spin laser can be commercially viable, it must be possible to excite the spin oscillations without another laser. This would involve injecting spin-polarized electrons into the laser – a process so far realized only at cryogenic temperatures. “Some of the advances that are now pursued in very different areas – such as magnetic hard drives and magnetic random-access memory – are based on better magnets and better methods of electrical spin injection,” concludes Zutic. “A broader view of these developments may allow useful transfer of knowledge.”
The work is described in Appl. Phys. Lett. 99 151107.