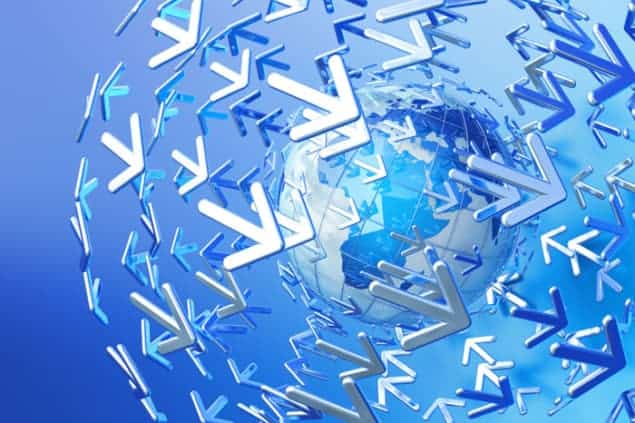
A new type of gyroscope based on interfering atoms has been developed that can determine the latitude where the instrument is located – and also measure true north and the Earth’s rate of rotation. The device has been developed by physicists in the US, who hope to scale it up so that it can test Einstein’s general theory of relativity. They also want to miniaturize the technology so it can be used in portable navigation systems.
The gyroscope has been built by a team led by Mark Kasevich at Stanford University in California. It works by firing a cloud of atoms upwards at a slight angle to the vertical so that the atoms follow a parabolic trajectory as gravity pulls them down. A series of laser pulses is then fired at the cloud while in flight, which separates the atoms into a number of different bunches that follow different trajectories. The pulses are carefully selected so that two of these trajectories cross paths at a detector.
Given that the atoms are governed by quantum mechanics, they behave like waves with a relative shift in phase between the atoms taking different paths. The resulting interference at the detector is dictated in part by the relative orientations of the laser pulses, gravity and the rotation of the Earth.
Where in the world?
The device is set up so that the laser pulses are fired horizontally – that is perpendicular to gravity – and was tested by rotating the orientation of the laser pulses about the gravitational axis. The resulting interference pattern is a near-perfect sinusoid with an amplitude that depends on the Earth’s rate of rotation and the latitude of the location where the measurement is made. Because we know how fast the Earth is spinning, the latitude can therefore be easily determined. The direction of true north and south are given by the direction of the laser pulses when the amplitude of the sinusoid is zero.
As the gyroscope is also sensitive to its own motion relative to its surroundings, Kasevich and colleagues have shown that it could be used for “inertial navigation”, whereby the location of a vehicle (or person) is calculated by knowing its starting point and all the movements that it has made. The team demonstrated this by rotating the gyroscope about the axis perpendicular to both gravity and the laser pulses, which led to a steady change in the interference as the angular velocity was increased from zero to about 1.6 revolutions per second.
Testing Einstein
Although this is not the first atom gyroscope to be made, the team says that its dynamic range is 1000 times greater than previous versions. Another important difference between this and other atom gyroscopes is that the interference pattern does not depend on the velocity of the atoms, which means that noise and uncertainty in those measurements do not degrade its performance.
Kasevich believes that the technique could also be adapted to measure – for the first time in a laboratory setting – the tiny corrections to the trajectory of any object resulting from Einstein’s general theory of relativity. “As our atom-interferometry technique essentially determines trajectory, ultimately, the interferometer phase shift should reflect those trajectory corrections related to general relativity,” he says. Kasevich and colleagues now plan to refine their technique so that it is sensitive enough to measure this effect, known as “geodetic precession”, and implement it in a 10 m “drop tower” that is being built at Stanford.
Although the “geodetic precession” of general relativity has previously been measured using instruments on board satellites, Holger Müller of the University of California, Berkeley thinks that “confirmation by atom interferometers would be received with great interest”. However, he warns that the implementing the upgrade experiment in the 10 m tower will be “a challenge”.
Kasevich also has plans to implement the technology in small devices that could be used in navigation systems – and indeed is already associated with a small company called AOsense, based in Sunnyvale, California, that plans to do just that. Kasevich told physicsworld.com that a device with a volume of just 1 cm3 could be useful for terrestrial navigation applications. The current experiment is contained within a cubic magnetic shield with sides that measure about 50 cm.
The research is described in Physical Review Letters.