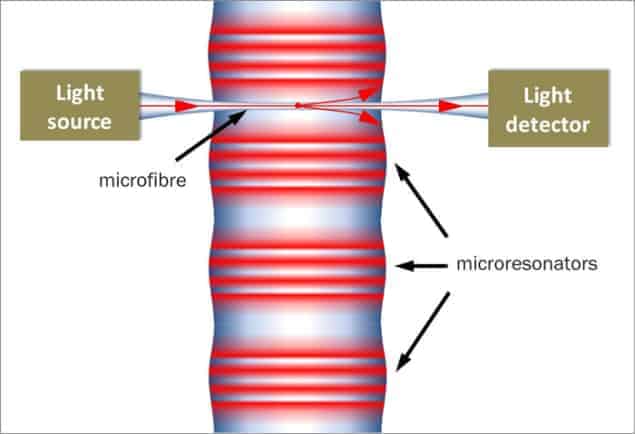
A tiny resonator that can store light without significant losses has been created by researchers in the US. The device is made from an ordinary optical fibre and is much more efficient than conventional resonators make from silicon. Such a low-loss, fibre-based resonator could boost the performance of telecommunications networks and could even lead to all-optical computers – claim the researchers.
Optical communication systems are extremely efficient when it comes to transmitting data in the form of light pulses. However, switching and processing data usually requires the pulses to be converted to electrical signals and then back into light again – operations that are costly both in terms of energy and chip size. Researchers are therefore keen on developing all-optical switches and processors, and one key challenge is how to store a light pulse for long enough for it to be manipulated.
One solution is an optical resonator, whereby a pulse is stored for a short period of time by having it bounce back and forth between mirrors. Silicon-based resonators can be made with dimensions of just a few hundred nanometres. However, according to Mikhail Sumetsky at OFS Laboratories in New Jersey, who was involved in this latest research, “The problem with [silicon] lithography is that it usually uses etching, and that causes roughness.” Because rough surfaces scatter more light than smooth ones, such resonators drop the signal at a rate of about 0.1 dB/cm as the light travels within them. In contrast, the resonator developed by Sumetsky and colleagues is shaped by heating and stretching an optical fibre, which gives it a much smoother surface and therefore a lower loss rate – less than 10–4 dB/cm.
Tugging with precision
The resonator itself is just a very slight bulge in the optical fibre, about 20–40 µm in diameter, which is created by heating points on either side of the fibre with an infrared laser and tugging the ends of the material so that the heated glass stretches and narrows. The narrow sections need only be about 10 nm slimmer than the bulges, and this method gave the team control over the diameter to a precision better than 0.1 nm.
John Fini, one of Sumetsky’s collaborators, says that the techniques used are similar to those employed for making microfibres years ago. “But an improved precision of two orders of magnitude enables us to fabricate these microresonators,” he adds.
In their experiment, light enters the resonator via a second optical fibre that runs from a light source, past the resonator, and finally to a detector (see illustration). The fibre narrows as it passes the resonator so that light can move easily between the two components. If the frequency of the light matches that of the resonator, the light is diverted into the resonator. Otherwise, the light travels past the resonator and on to the detector.
Spiralling round the bulge
Once inside the resonator, the light spirals along the surface of the bulge until it reaches a constriction, where it is reflected back. The result is a trapped light wave with a pattern of intensity peaks and nodes within the bulge.
With a single resonator, the researchers say that they trapped light for 100 times longer than is possible with lithographically made resonators. Furthermore, they claim that they could hold light even longer by adding more resonators. This is as simple as heating more points on the fibre and stretching, and the team has demonstrated a series of 10 resonators on a single optical fibre. The neighbouring resonators can pick up the light before it heads toward the detector, thus storing it for longer periods.
Uday Khankhoje of NASA’s Jet Propulsion Laboratory in Pasadena, California, suggests that these microresonators could be a good option for routing optical data on the Internet. A router holds data until the path is clear for them to travel on, a function known as buffering. In traditional routers, optical data would be converted to electronic signals for storage and direction, then reconverted to optical signals later. “As these new devices are made of optical fibres themselves, and the reported losses are quite low, it would be practical to use their light-storing functionality to buffer light,” says Khankhoje.
Shuttling light a problem
Khankhoje is sceptical, however, of the application of the new resonators in optical computing. “A very serious practical issue is that a lot of optical power would be lost in shuttling light between these devices and lithographically fabricated devices; the latter being required to perform core computational tasks,” he says. Sumetsky argues that a very low-loss connection between lithographically made waveguides sitting on top of the optical fibres has already been demonstrated, and that the resonators should work in a similar system.
Takasume Tanabe of Keio University, Japan, notes that although other microresonators are capable of storing light for longer times, this technology stands out for practical applications because the resonators are made with common optical fibre, and the team has shown that the devices can be connected. “Combining more than one cavity brings the possibility of having slow light for memory buffering, enhanced nonlinearity for all-optical logic operation, and fibre sensing,” he says.
This research is described in papers published in Optics Letters and Optics Express.