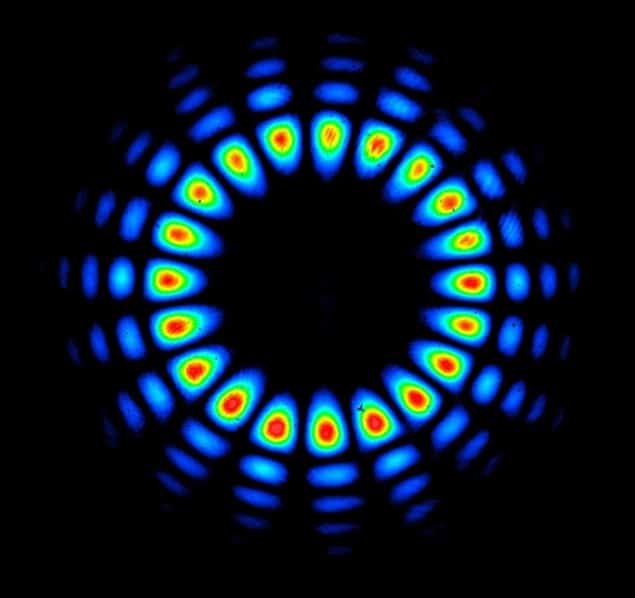
Physicists in Austria have devised a new technique for entangling photons using the property of “orbital angular momentum”. The researchers say that the large amount of orbital momentum they have imparted to the photons paves the way for the entanglement of macroscopic objects and could also find applications in remote sensing and quantum computing.
Entanglement is a connectedness between two (or more) particles that does not exist in classical physics. It means that determining the quantum state of one of the particles automatically and instantaneously fixes the quantum state of the second particle, no matter how far apart those particles are – a phenomenon that Einstein famously called “spooky action at a distance”. Often this is achieved using the polarization of photons – the direction of vibration of a light wave’s electric field – such that pairs of entangled photons are constrained to vibrate at right angles to one another even though each of the photons is randomly polarized.
Entangled pairs
In the latest research, Anton Zeilinger, Robert Fickler and colleagues at the University of Vienna entangled photons in orbital angular momentum (OAM). Giving photons OAM means twisting a beam’s wavefront so that as the beam travels forward its wavefront rotates around the propagation axis. This property has been well studied using laser beams and is exploited in so-called optical spanners, which use lasers to trap and rotate small objects. But Zeilinger’s group was interested specifically in entangling twisted photons; in other words, producing pairs of photons with opposite directions of twistedness. That twistedness is represented by the quantum number l – the number of times the wavefront rotates around the propagation axis in the space of one wavelength. “The goal of our experiment was to see how high we could get this number,” says team member Radek Lapkiewicz.
Other groups have previously entangled photons with OAM by firing laser beams into “nonlinear” crystals and then siphoning off the very small fraction of photons that spontaneously split inside the crystal to produce two lower-energy entangled photons. Those entangled photons carry a broad spectrum of OAM. But, says Lapkiewicz, this approach, being “limited by what nature gives”, yields l values only up to about 20.
Twisting around
The Austrian group also used a nonlinear crystal to generate photons. In this case, however, the photons were entangled in polarization and this entanglement served only as a first step. The next step was to send the photons within each pair down separate optical fibres and then impart them with OAM. The researchers did this by bouncing the photons off a tiny screen known as a spatial light modulator, which is a device that alters the phase of the reflected light from point to point, so changing the shape of the beam’s wavefront. This wavefront deformation depended on the photons’ polarization, so that photons polarized in one direction received a kick of positive OAM whereas those polarized at right angles got a negative kick. The net result was to change the photons’ entanglement from one of polarization to one of OAM.
Using this technique, Zeilinger and co-workers found they could obtain differences in quantum number as high as 600 (in other words l = +300 on one photon and l = –300 on the other). Lapkiewicz points out that there is, in theory, no upper limit to a photon’s l value, which suggests that a photon – a quantum object – could acquire as much OAM as a macroscopic object, leading to what he calls a “tension between the quantum and classical worlds”. But he cautions that the current result is still “many orders of magnitude” too small to rotate even tiny objects. He speculates that such manipulation might one day be possible by combining the momentum of many photons entangled together.
Remote senses and quantum information
Zeilinger’s group also says that its technique could be useful for carrying out remote sensing, particularly in low-light biological-imaging experiments. The idea would be to measure tiny rotations by attaching the rotating object to a circular mask with regularly spaced radial slits. One photon in each entangled pair would be given a high OAM value, while the other would keep its polarization. With the mask placed in the path of the OAM photons and rotated very slightly, the rate of simultaneous detection of the two sets of photons would change. The trick is that a polarizer placed in the path of the polarized photons would need to rotate through l times as large an angle to register the same change, so multiplying the sensitivity of the measurement by l times.
According to Lapkiewicz, the work might also be applied to quantum information. For example, he says, it might allow quantum processors that rely on polarization entanglement to be connected to those that instead exploit OAM.
Hans Bachor of the Australian National University believes that OAM entanglement “will have profound implications for both the communication of quantum information as well as quantum-logic protocols”, and he says that Zeilinger’s group has taken “an important step ahead” in this field. But he argues that it will be crucial to demonstrate entanglement with many modes. “The work here shows entanglement between two modes with widely different quantum numbers,” he adds. “That is important, but it is still only the entanglement between two modes.”
The work is published in Science.