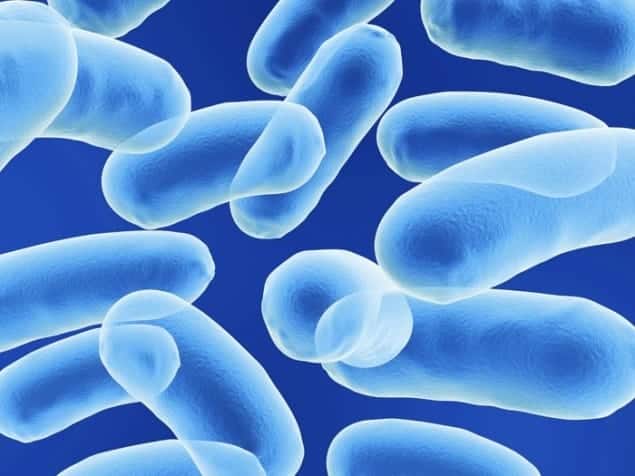
The Casimir effect is perhaps best known as a quantum phenomenon, in which vacuum fluctuations can give rise to an attractive force between two parallel mirrors. But there is also a thermodynamic equivalent, caused by fluctuations in the composition of a fluid close to its critical point. New research by physicists in the US suggests that these “critical Casimir” forces act on the proteins inside cellular membranes, allowing proteins to communicate with one another and stimulating cells’ responses to allergens such as pollen.
All cells are surrounded by a membrane that controls the flow of substances into and out of the organism. Membranes are made up of molecules called lipids within which proteins are embedded. They were once thought to be essentially uniform, but a number of experiments starting in the 1970s and 1980s indicated that the lipids in fact cluster to form distinct structures tens or hundreds of times larger than the lipid molecules themselves. Scientists did not understand, however, where the energy needed to maintain such structures came from.
In 2008 biophysicist Sarah Veatch at Cornell University in upstate New York and colleagues found a solution. It was known that above 25 °C membranes isolated from live mammal cells exist in a single liquid phase, whereas below that temperature they separate out into two distinct phases, composed of different kinds of lipids and proteins – a bit like oil and water refusing to mix when brought together. What Veatch’s group discovered was that as they lowered the temperature of the membranes close to that at which the phases separate out, known as the critical point, small fluctuating patches of the second phase started to appear. Such fluctuations – which measured several microns across and were visible in an optical microscope – do not require large amounts of energy to form.
Critical look at criticality
Veatch has since moved to the University of Michigan but for the current research teamed up with two physicists back at Cornell, Benjamin Machta and James Sethna, to understand the purpose of this criticality. The researchers reckoned that certain kinds of proteins are attracted to one of the phases while other kinds are attracted to the second phase, so tending to draw like proteins together and separate out unlike proteins. As Veatch explains, these interacting proteins would form “signalling cascades” to transmit information regarding the identity of compounds in a cell’s vicinity from receptor proteins in the membrane to the inside of the cell. Such information could be used, for example, to decide whether it is a good time to divide or whether it is safe to crawl towards food. “We think that one reason cell membranes contain critical fluctuations is to help facilitate some of the early steps in these signalling pathways,” she says.
To calculate the strength and form of the Casimir forces between proteins, Machta used mathematics developed originally for string theory. He found that, as expected, the forces are attractive for like proteins and repulsive for unlike ones, and that they yield a potential energy several times that of the proteins’ thermal energy, over distances of tens of nanometres. Much stronger electrostatic interactions, he explains, are limited to ranges of about a nanometre by the screening effects of ions inside the cell. “We have found that by tuning close to criticality, cells have arranged for a long-ranged force to act between proteins,” he says.
Sethna adds a broader perspective. “It is amazing how many reactions in cells all involve energies of the same size as thermal fluctuations,” he says. “We think that it is the cell being economical – why pay more?”
Something to sneeze at
The researchers suspect that the existence of these critical Casimir forces explains why cells low on cholesterol do not function as they should – the removal of the cholesterol, they reckon, taking the membrane away from its critical point. They also speculate that the forces are involved in the sneezing process. Sethna explains that when the receptor proteins in immune cells detect an allergen such as pollen they cluster together, and this clustering somehow triggers the histamines that cause sneezing. He says that perhaps an allergen simply changes the preference of the receptor proteins for one of the two liquid phases in the membrane, hence drawing them together.
The team is hopeful that its work could lead to medical applications. Veatch explains that defects in lipids are thought to contribute to a large number of diseases, including cancer, auto-immunity diseases, and inflammation. “This work may shed light on how lipids could impact some aspects of these diseases,” she says. “In the future, I can imagine drugs that specifically target lipids to regulate interactions between proteins in order to treat human disease.”
Sethna adds, however, that the time scale for such applications is likely to be long. “Our work is more like figuring out how to make better concrete to build the subbasement of the skyscraper that eventually would house the penthouse of health applications,” he says.
Theory explains behaviour
But in addition to any future applications, Sethna argues that the existence of criticality within cells lessens the reliance on purely evolutionary mechanisms when trying to understand how cells operate. “There are lots of things about cells that biologists assume happen because ‘evolution made it so’,” he says. “Here, I guess, evolution allowed the cell to find this critical point. But once the cell is at the critical point, we can use systematic, cool theory to explain lots of the behaviour, without repeatedly accounting for everything using evolution.”
However, some independent experts feel that Veatch’s experimental results must be treated with caution because they were not obtained using intact cells. One, who asked to remain anonymous, argues that the separation of the membrane from the rest of the cell might have removed certain relevant components from the membrane and that the body of the cell itself might influence the critical fluctuations in some way. “I am not yet convinced that the theory presented is applicable to in vivo biological membranes,” he says. “I therefore think that much more experimental work has to be done to investigate this phenomenon.”
The research is described in Physical Review Letters.