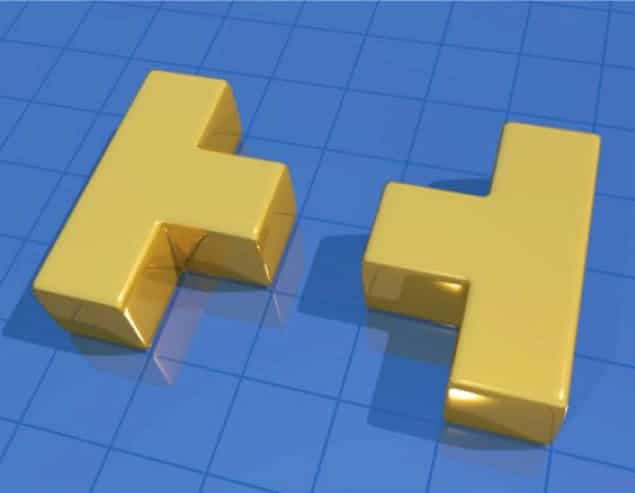
Physicists in Germany have used evolutionary algorithms to help them pinpoint the best geometry for a nanoantenna. As well as zeroing in on the optimal design out of more than 10132 alternatives, the technique has provided unexpected new insights into the complex optical properties of nanostructures.
Nanoantennas convert light to electrical power and vice-versa, and are essential in the design of tiny electro-optical devices. They have diverse potential applications in just about anything based on light–matter interaction, including optical sensing and signalling, microscopy, solar-power conversion and quantum cryptography.
An antenna’s ideal size is dictated by the wavelength of the radiation that it handles. For radio waves, this is of the order of metres, and for light it is hundreds of nanometres. Most research so far has focused on designs that are essentially miniaturized radio antennas. However, tiny metal components interact with light in a much different way from how radio waves interact with larger components – and this means that shrinking radio designs is not necessarily the best approach to take.
Employing evolution
Instead, Thorsten Feichtner and colleagues of the University of Würzburg have used evolutionary optimization to wheedle-out the most structurally well adapted nanoantenna for their purposes. Inspired by natural selection, evolutionary optimization algorithms work towards an ideal design rather than evaluating the performance of all possible designs. For the problem tackled by Feichtner’s team, the latter would be impossible because more than 10132 antenna designs would need to be evaluated using a process that takes 20 minutes per structure.
The team’s goal was to find a geometry that would enhance the near-field intensity of an illuminating beam of light as much as possible, so they chose this as the “fitness parameter” that they would judge each design against. Their antennas consisted of 21 by 21 matrices of gold cubes measuring 10 nm on the side. Beginning with patterns that were generated randomly, they ran simulations on batches of 20 designs to establish which were best adapted to the design goal.
Just as in nature, the fittest patterns got the chance to pass on their characteristics to the next generation, while the weaker specimens were discarded. The highest-performing five from each batch were used to build a new generation of 20 structures via crossing techniques and mutations. The new structures were in turn pitted against one another, so the overall fitness of the designs improved generation by generation – over 100 generations – until the near-field intensity enhancement registered almost twice that of the reference antenna.
Puzzling patterns
“In the end, we found that the best shape was a very random-looking pattern of gold blocks,” says Feichtner. The central part of that design was reminiscent of a classical antenna though: two rod-like structures separated by a gap. When the researchers took a close look at the currents flowing in that area, they discovered that the rods were connected by a single gold block, positioned just below the gap between the rods.
“If you look at the problem purely geometrically, you should have no conductive link between two blocks which are just touching at the edges. But the simulation approach we used allowed current to flow along the edges from one cube to another,” explains Feichtner. “This was a bit of a surprise to us … and when we removed just this one block, the fitness decreased by a factor of two.”
“Of course, this whole structure is very complex and we’re far away from understanding what is happening everywhere,” he adds. Rather than pursue that answer too doggedly, the team instead came up with a stripped-down version of their fittest candidate, which might feasibly be built with today’s technology – essentially a hybrid of a two-wire antenna and the split-ring resonator geometry that they discovered – and showed that it, too, boasted the same two-fold increase in fitness factor.
Hot topic
This is certainly not the first study to demonstrate that nanoantennas can be optimized using evolutionary algorithms but,” says Feichtner, “[previous studies] were restricted to changing the shape of one particle, or using a defined-shape particle and moving them round or changing the size. Our approach allows one to build up arbitrary geometries made of one or multiple particles, and also gives the possibility to see how large connected structures will work. The split-ring geometry would never have been found with the approaches that have been used thus far.”
The study indeed “introduces a very original way of designing optical antennas”, according to Sébastian Bidault, who investigates DNA-based fabrication of nanoantennas at the Institut Langevin in Paris, France, and was not involved in the research.
He points out, however, that one of the main drawbacks of metal-based nanoantennas is strong losses due to local heating. “Optimizing local intensity enhancement by adding more metal that will induce more ohmic losses [fails to] consider what happens to this energy afterwards.”
The research appears in Physical Review Letters.