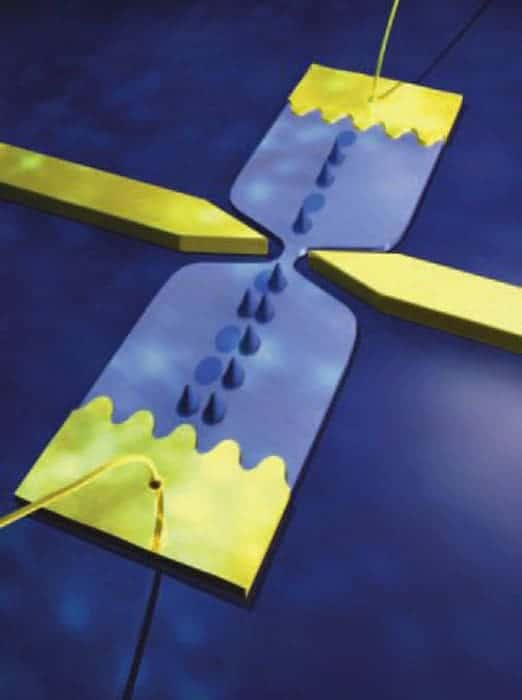
A new type of quasiparticle – dubbed the “leviton” – has been seen by physicists in France and Switzerland. First predicted in 1996 by a team led by Leonid Levitov, the phenomenon involves the excitation of as few as one electron to create a wave that propagates coherently through a metal. The ability to make levitons on demand could lead to the creation of quantum-electronics circuits that involve sending single electrons through tiny circuits.
Electrons in a metal or semiconductor can be thought of as a “Fermi sea” of particles, with the highest-energy electrons at the surface. Normally, if an electron receives an extra kick of energy it pops out of the Fermi sea, creating a “hole” – which is itself a quasiparticle. However, under special circumstances an electron (or a few electrons) can rise out of the Fermi sea without creating a hole – much like a wave rising out of the ocean. This excitation could then propagate through the material like a tiny particle that obeys quantum mechanics – a quasiparticle.
Searching, searching, searching
After this type of excitation was first suggested by Levitov and colleagues, it immediately inspired another physicist, Christian Glattli, who has been trying to devise experiments to create levitons ever since. Working at CEA Saclay near Paris, Glattli’s team – along with physicists at the University of Paris Diderot and ETH Zürich – has now succeeded in creating levitons and confirming their existence using several different techniques.
Their experiments were carried out on a metal film that is so thin that its electrons behave like a 2D gas. Levitons are created at one end of the device using an electrode that applies an electrical pulse with a specific temporal shape – the familiar Lorentzian distribution. The levitons then travel through a quantum point constriction (QPC) that is created in a narrow gap between two electrodes that are halfway along the device (see figure). When an appropriate gate voltage is applied to these electrodes, the gap becomes a 1D channel for electrons. After negotiating the QPC, the charge carried by the levitons is detected using a fourth electrode at the far end of the device.
Listen to the noise
While the arrival of an electron pulse can be measured at the fourth electrode, the researchers cannot tell if it was carried by a leviton or via a more conventional electron–hole excitation. So to confirm that levitons are created, the team used a technique called noise spectroscopy, which involves cooling the device to a chilly 35 mK and measuring the electronic noise in the sample.
Theory says that there should be more noise in the sample when electron–hole excitations are present than when levitons are there. The team therefore measured the noise when Lorentzian pulses are applied to the device. Measurements were also made using square and sinusoidal pulses because these are more likely to produce electron–hole excitations, rather than levitons. Sure enough, there was significantly less noise when Lorentzian pulses were used, compared to square and sinusoidal pulses.
Electron anti-bunching
To further confirm that the levitons are indeed quasiparticles that obey the rules of quantum mechanics, the team did a “Hong–Ou–Mandel experiment” whereby two levitons are fired at a beam splitter at the same time from opposite ends of the device. The QPC acts as the beam splitter and, because levitons obey Fermi–Dirac statistics, two levitons will always follow different paths through the beam splitter. This is a quantum-mechanical effect called anti-bunching.
Again, Glattli and colleagues used noise spectroscopy to look for evidence of anti-bunching. It turns out that when anti-bunching occurs, the noise associated with the levitons should vanish. The team confirmed this by adjusting the time delay between the levitons reaching the beam splitter and measuring the noise. As the time delay went through zero, anti-bunching occurred and the noise decreased significantly, just as expected for levitons.
According to Glattli, the levitons have the same effective mass as electrons and interact with electromagnetic fields in the same way. As a result, the leviton source can be thought of as a source of single electrons that operates on demand – rather than emitting electrons at random times. Such sources have proven to be difficult to build and could have important applications in quantum computing and quantum metrology that use single electrons in much the same way that quantum optics systems use single photons.
‘Significant step forward’
JT Janssen, who develops quantum-metrology techniques at the UK’s National Physical Laboratory (NPL), described the work as “a significant step forward”. He highlights the fact that Glattli and colleagues were able to show that the levitons remained coherent over a distance as great as 100 μm – something that is important for practical applications.
Leonid Levitov told physicsworld.com that Glattli’s work is “complete and convincing”, adding that the detection technique is similar to that outlined by Levitov and colleagues in a paper published in 2006. Levitov, who works at the Massachusetts Institute of Technology, said that he is proud of the work that he did in the 1990s and is pleased that it has been confirmed in the lab. “As for the playful name, I think it’s alright as long as it helps to convey the message, which it probably does.”
As for Glattli, he is currently working with a group that specializes in creating ultracold gasses of fermionic atoms to see if levitons can be created in such systems.
The research is described in Nature.