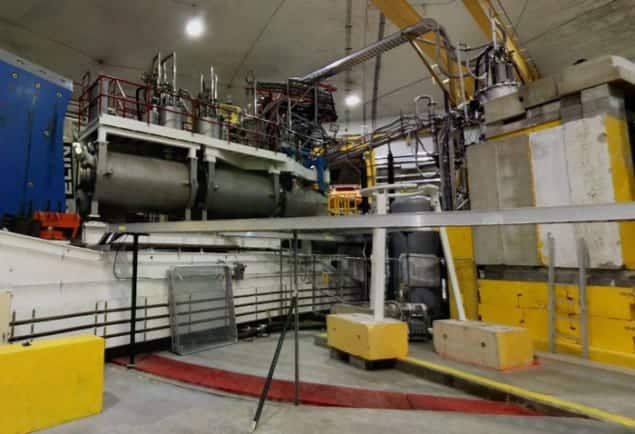
An international collaboration has made the first determination of the proton’s “weak charge” – a quantity that is related to the strength of the weak interaction. The Q-weak experimental collaboration, working at Jefferson Lab in Newport News, Virginia, says that the small number of data analysed so far agree with predictions of the Standard Model of particle physics but that it believes a full analysis could still reveal the existence of “new physics”.
All the fundamental forces in nature have a strength that is determined by a certain particle parameter. For instance, the strength of gravity is determined by particle mass, while the strength of electromagnetism is determined by electric charge. Similarly, the strength of the weak force, which governs radioactive decay, is determined by a property known as weak charge. In fact, physicists have known that the electric and weak charges are related to one another since the late 1960s, when the electromagnetic and weak forces were unified into a single electroweak theory, which forms part of the Standard Model of particle physics.
Precise measurements of weak charge go back several decades, when the property was determined for caesium-133 nuclei. More recently, in 2004, physicists at the Stanford Linear Accelerator Center in California measured the weak charge of the electron and found it to be consistent with the Standard Model prediction of slightly greater than zero. The weak charges of the electron and proton are particularly interesting because the particles are relatively simple systems. This simplicity is important when it comes to interpreting any measured deviations from Standard Model predictions.
Challenging measurement
In the past, measuring the proton’s weak charge has been considered almost too challenging to perform. One problem is that the proton is not a point-like particle – it is made up of three quarks. As a result, theorists have needed to make sure that they fully understand the contributions from its internal structure. But another problem is experimental: the measurement requires an incredibly powerful and stable electron beam in which all of the electron spins are polarized in the same direction. This beam must be fired at a proton so that deviations in scattering for different polarizations as subtle as a few parts in a billion can be recorded.
Such challenges have now been met by the Q-weak experimental collaboration. Using the particle accelerator at Jefferson Lab, the researchers sent a beam of spin-polarized electrons into a sample of liquid hydrogen – essentially, a flask of protons – so that the electrons scattered off at small glancing angles could be measured. Theory says that the proton’s weak charge has a subtle influence on the rate at which the electrons are scattered. This effect is also dependent on the spin of the electrons; so by flipping the electron spin and recording the change in scattering rate, the researchers were able to determine the weak charge of the proton.
The Standard Model predicts very precisely that the weak charge of the proton ought to be almost zero. This is what the Q-weak collaboration measured – but having analysed just 4% of the data. “Look at this primarily as the first determination of the weak charge of the proton, not as our final test of the Standard Model,” says Roger Carlini, a spokesperson for the collaboration. He expects a more definitive result in about a year. “The jury is still out!” he adds.
Surprises lurking in the data
Indeed, Carlini is open to the suggestion that physics beyond the Standard Model could still show up in the final results as he and his colleagues analyse the rest of the data. Such new physics might be particles that are far heavier than anything that is currently known. For instance, heavier particles are predicted in supersymmetry, a theory that partners all the current elementary particles with a host of more massive “sparticles”. They are also predicted by so-called grand unification theories, which aim to unify the strong force with electroweak theory.
If beyond-the-Standard-Model physics has so far been eluding us in this stealthy way, the weak-charge measurements might provide the first indications that it is there
Michael Ramsey-Musolf, University of Massachusetts-Amherst
Carlini admits that any evidence of new physics in a more thorough measurement of the proton’s weak charge will not be direct, as it might be for instance at the Large Hadron Collider (LHC) at CERN in Geneva, Switzerland. “If you see new physics in an LHC experiment, you have it in the bag, so to speak,” he says. But he adds that the LHC can still run out of energy before it detects anything – a handicap that is not suffered by a “precision frontier” experiment such as Q-weak. “We don’t have to build higher and higher energy accelerators, just conduct more and more precise measurements. Which in the end may be easier,” he says.
Indeed, theorist Michael Ramsey-Musolf at the University of Massachusetts-Amherst believes that certain parts of theories, including supersymmetry, would never show up at the LHC but have the potential to show up in weak-charge measurements. “If beyond-the-Standard-Model physics has so far been eluding us in this stealthy way, the weak-charge measurements might provide the first indications that it is there,” he says.
Theorist Victor Flambaum at the University of New South Wales in Sydney, Australia, agrees that the measurements will provide an important test of supersymmetry and grand unification theories. “The Jefferson Lab measurement of the proton’s weak charge is a great achievement,” he adds.
The research is due to be published in Physical Review Letters. A preprint can be found at arXiv:1307.5275.