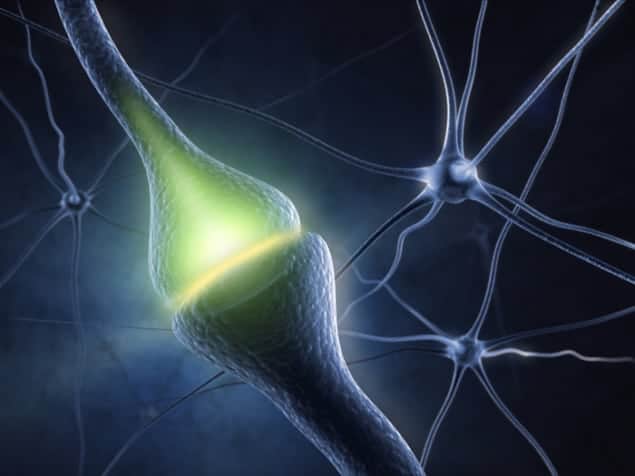
Researchers in the US have for the first time used MRI to follow the dynamics of neurotransmitters with molecular precision. They have demonstrated the technique on dopamine, a neurotransmitter that represents processes of reward and motivation in the brain.
Neurotransmitters are the chemicals released at the end of nerve fibres to communicate signals to other nerve fibres in the vicinity. An understanding of their dynamics is important for a more general understanding of brain function, yet they are hard to study. In the past, scientists have resorted to PET, an imaging technique that relies on a radioactive tracer being inserted into the body so that its path can be monitored by emitted gamma rays. But PET can only supply images that are spatially accurate to within a few millimetres and temporally accurate to within a few minutes.
Contrasting agents
Functional MRI (fMRI) could be more precise. As in standard MRI, fMRI involves the use of a fixed magnetic field to align the spins of protons inside the water molecules of tissue. After radio waves have deflected these spins, their relaxation back to alignment is timed with a radio receiver coil, and this time reveals the tissue’s composition. The difference with fMRI is that paramagnetic molecules known as “contrast agents” interact with some of the water molecules, thereby changing their brightness in scans. Haemoglobin in blood is a natural example: on its own, it acts as a contrast agent, but when it is bound to oxygen, its effect is diminished. In this way, scientists can use fMRI to study the circulation of oxygenated and deoxygenated blood.
Now, medical engineer Alan Jasanoff and colleagues at the Massachusetts Institute of Technology (MIT) in the US have experimented with another contrast agent – the paramagnetic haem protein BM3h. Instead of turning off when bound to oxygen, BM3h turns off when bound to dopamine, and can therefore be used with fMRI to sense the progression of the neurotransmitter between nerve fibres. The team claims that this form of imaging is an order of magnitude more accurate than PET. “Our study is the first to use MRI to study the dynamics of neurotransmitter release and signalling,” says Jasanoff.
The MIT group tested its technique on live rats by injecting BM3h into a region of the brain known as the ventral striatum, which emits dopamine, and electrically stimulating the medial forebrain bundle (a part of the reward system). Each dopamine stimulation lasted 16 s and the researchers took an MR image every 8 s, allowing them to track how dopamine levels changed as the neurotransmitter was released from cells and then disappeared.
Dopamine dynamics
“Neurotransmitters play distinct functional roles, which we can study by imaging their dynamics,” says Jasanoff. “For instance, dopamine is important for the brain’s ‘internal representation’ of reward and motivation, and if we want to figure out how dopamine works to achieve this, we should learn where and when dopamine concentrations are changing in the brain.”
The researchers found that an area known as the nucleus accumbens core (NAcC), which is known to receive dopamine from an area of the brain, showed the highest levels of dopamine release, according to the fMRI scan. They also found that dopamine was released in neighbouring regions such as the ventral pallidum, which regulates motivation and emotions, and parts of the thalamus, which relays sensory and motor signals in the brain.
Jasanoff and colleagues also believe that the technique could help in studies of Parkinson’s disease, which is caused through death of dopamine-generating cells. But Jasanoff is not going to stop with the imaging of dopamine by fMRI. “We are also developing and applying molecular sensors for MRI-based mapping of many other aspects of neural activity,” he says.
The research is published in Science.
- This article first appeared on medicalphysicsweb.org.