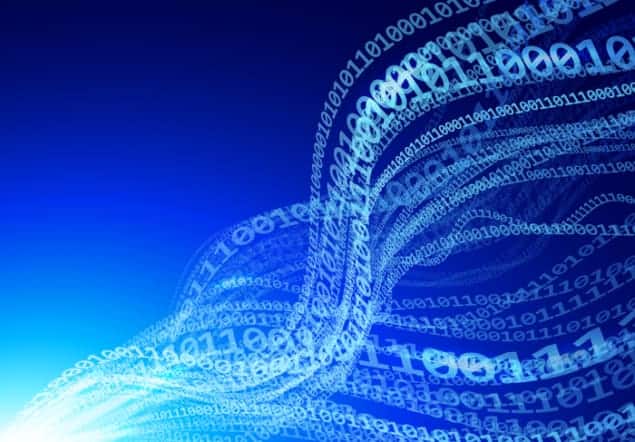
A way of modulating the waveforms of individual, coherent high-energy photons at room temperature has been demonstrated by researchers in the US and Russia. The advance opens the way for new quantum-optics technologies capable of extremely high-precision measurements, as well as the possibility of quantum-information systems based on nuclear processes. The new approach could also be useful for those doing fundamental research in a variety of areas, ranging from the role of quantum phenomena in biological processes to fundamental questions in quantum optics itself.
The technique was developed by Olga Kocharovskaya, Farit Vagizov and colleagues at Texas A&M University and the Kazan Federal University. Their set-up bears some similarity to a Mössbauer spectroscopy experiment. A sample of radioactive cobalt-57 decays to an excited state of iron-57, which then decays by emitting a 14.4 keV “soft” gamma-ray photon. This photon can then be absorbed and re-emitted by a nearby stainless-steel foil containing iron-57. Because of the Mössbauer effect, no energy is lost in the recoil of the stainless-steel lattice and the photon is emitted at 14.4 keV with very little spectral blurring.
As the foil absorbs and re-emits the photons, it is vibrated at megahertz frequencies. By making clever use of the Doppler effect, the team is able to shape a single photon into a double pulse and even a train of ultrashort pulses. This makes it possible to use the gamma-ray photons to encode quantum information in a “time-bin qubit” – quantum bits in which information is encoded in terms of the relative arrival time of pulses.
Easier to detect
The new method offers advantages over schemes for encoding quantum information in lower-energy optical or microwave photons. Photons in the 10–100 keV energy range – soft gamma rays from nuclear transitions and “hard” X-rays from atomic transitions – penetrate deeper into materials and can be detected with greater efficiency. And in the case of the Mössbauer photons, the recoil-less nature of the effect means that the re-emitted photons retain their quantum coherence at room temperature.
Recent developments towards the generation of entangled gamma-photon pairs, combined with the team’s new method, could lead to quantum-computing applications that use nuclei as qubits. “Entangled nuclear ensembles may be produced via resonant interactions with the entangled photons, using quantum-memory protocols,” explains Kocharovskaya.
The new technique gives physicists a more versatile way of controlling gamma rays than current methods of manipulating high-energy photons. “In our approach, we have more parameters to manipulate,” Kocharovskaya says. Indeed, the team can alter the amplitude, frequency and phase of the radiation and change the nature and temporal profile of the modulating wave. Arbitrary waveforms can be created “on demand” by changing the absorber depth and frequency of resonance. “Besides, our method allows one to manipulate single gamma-photons, which is impossible with the existing nonlinear techniques,” she says. Kocharovskaya and colleagues are now working to improve their technique as well as pursuing a number of potential applications.
Deterministic pulse shaping
“This study opens for the first time possibilities for deterministic pulse shaping in the X-ray regime,” says X-ray optics expert Ralf Röhlsberger of DESY in Hamburg, Germany. “Unfortunately, the positive aspects come with some drawbacks.” He points out that photons in this energy range are difficult to manipulate using conventional optical devices and that photon loss through absorption and scattering will limit performance. “These technologies, however, have nevertheless the potential to boost our understanding of fundamental aspects of the light–matter interactions,” he says.
Christoph Keitel of the Max Planck Institute for Nuclear Physics in Heidelberg, Germany, agrees that the work of Kocharovskaya and colleagues is a significant accomplishment. He points out that “this is a demanding field with numerous serious challenges: the radiation sources are weak and often relatively dirty, and the couplings to the nuclei are generally small. This renders this absolutely clean and innovative way of coherently adapting gamma waves so impressive.”
The technique is described in Nature and a preprint is available on arXiv.