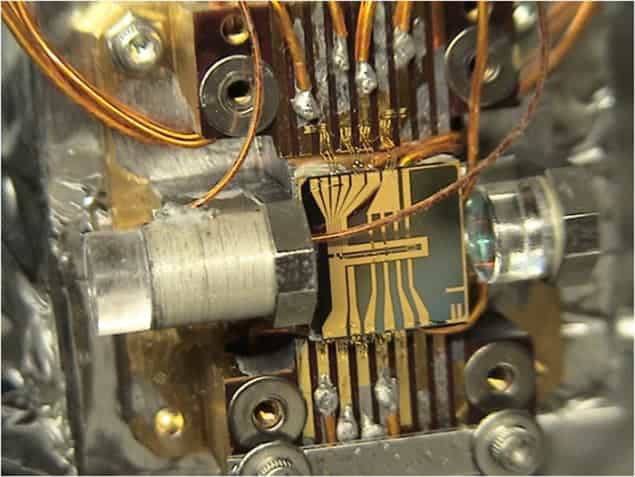
Physicists in the US and Serbia have created an entangled quantum state of nearly 3000 ultracold atoms using just one photon. This is the largest number of atoms ever to be entangled in the lab, and the researchers say that the technique could be used to boost the precision of atomic clocks.
Entanglement is a purely quantum-mechanical phenomenon that allows two or more particles to have a much closer relationship than is allowed by classical physics. One property of entangled particles is that they can be very sensitive to external stimuli such as a gravity or light, and therefore could be used to create precise “quantum sensors” and clocks.
Until this latest experiment by Vladan Vuletić and colleagues at the Massachusetts Institute of Technology and the University of Belgrade, physicists had managed to entangle about 100 atoms within a much larger ensemble of atoms. Now, Vuletić’s team has managed to entangle nearly 94% of the atoms in its ensemble of 3100 atoms.
The experiment involves an optical cavity – two opposing imperfect mirrors – containing about 3100 rubidium-87 atoms that are cooled to a temperature of near absolute zero. Light is shone into one side of the cavity and allowed to bounce back and forth between the mirrors. Some of the light will eventually escape through the opposite side of the cavity, where it is captured by a detector. A magnetic field is applied to the atoms, which causes them to align their spins along the length of the cavity. However, the probabilistic nature of quantum mechanics means that the spins are not all aligned and their directions will fluctuate about the magnetic field.
Preliminary experiment
These fluctuations were measured in a preliminary experiment that involves firing a pulse of polarized light into the cavity. The pulse interacts with the atomic spins and emerges from the cavity with a small change or rotation of its polarization. This rotation is a measure of the direction of the total atomic spin of the gas relative to the direction of the magnetic field. By making this measurement many times over, the team showed that the total atomic spin in the cavity has a Gaussian distribution that is a disc centred on the direction of the applied magnetic field.
This representation of the total spin is called a “Wigner function” and it illustrates how the probabilistic nature of quantum mechanics causes fluctuations in the direction of the total spin. However, the fact that the function is Gaussian means that the atomic spins are behaving independently of each other and are not entangled. Therefore, the challenge for the researchers was to prepare the atoms in such a way that the Wigner function becomes non-Gaussian – which is strong evidence for entanglement.
To do this they fired an extremely weak polarized laser pulse into the cavity. Occasionally, just one photon in the pulse will bounce back and forth in the cavity and interact with nearly all of the atomic spins. This succession of interactions is what entangles the atoms.
Heralding entanglement
This photon can then leave the cavity and be detected. Such entangling photons are identifiable because their polarizations have been rotated by 90° by the atomic interactions. So, whenever such a “herald” photon was detected, the physicists immediately measured the direction of the total atomic spin. They repeated this process many times over to determine the Wigner function of the entangled atoms. Instead of a Gaussian disc, the distribution resembled a ring of positive probability surrounding an inner region of negative probability.
According to Vuletić, this hole of negative probability is the hallmark of entanglement. Furthermore, the researchers were able to calculate that the entanglement involved about 2910 of the 3100 atoms. In this experiment, the atomic spins were entangled in two states that lie on opposing sides of the hole of negative probability.
Vuletić says that the research has important practical applications because the Wigner function is essentially a measure of the uncertainty in a quantum measurement. By entangling large numbers of atoms and effectively “punching a hole” in the centre of the Wigner function, the precision of a quantum measurement can be improved. He told physicsworld.com that his team is now using the entanglement technique to create a more precise atomic clock.
The experiment is described in Nature.