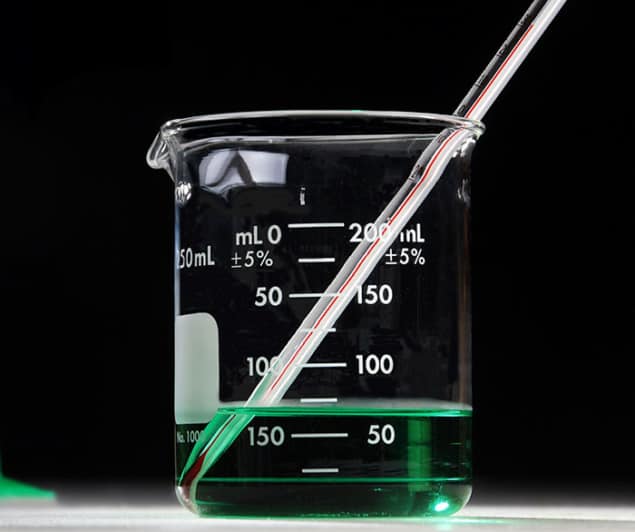
A special type of laser spectroscopy has been used by researchers in Australia to measure the velocities of atoms in caesium vapour. The technique could allow researchers to infer both the temperature of the vapour and the lifetimes and energy separation of the atomic states. It can also be used to measure Boltzmann’s constant, thereby helping to redefine the kelvin relative to universal physical processes.
Today, the temperature of an object in kelvin is defined relative to the triple point of water – the point at which ice, liquid and steam exist in equilibrium. In 2018 the kelvin is to be redefined in terms of the physics underlying temperature, which is “fundamentally a measure of the energy of the atoms and molecules in an object”, says metrology expert Michael de Podesta of the National Physical Laboratory in Teddington, UK. “We’re going to specify a value of Boltzmann’s constant, which is a certain number of joules per degree. That will tell you fundamentally that, if an object has this much energy of motion, then its temperature is this.”
Transition widths
Several groups are attempting to measure Boltzmann’s constant in different systems. The best measurement to date has an uncertainty of less than one part per million. It was made in 2013 by a team led by De Podesta, who used the speed of sound in argon gas to deduce the constant. In the new research, a team of physicists at several Australian universities used a different technique called Doppler-broadening thermometry, which relies on the spectral width of specific atomic transitions.
The researchers focused on two absorption lines in the caesium spectrum, corresponding to the same atomic transition but separated by the hyperfine splitting of the excited states. These lines are broadened by two underlying effects: the intrinsic uncertainty of the state’s energy as defined by Heisenberg’s uncertainty principle – which leads to a Lorentzian distribution – and the fact that, if an atom is moving towards or away from the laser, it sees a Doppler-shifted laser frequency. This latter effect provides a Gaussian variation in the frequencies to which the laser responds. The hotter the sample becomes, the faster the particles are moving, so this Doppler shift becomes more significant and the peaks become broader. By measuring the relationship between peak width and temperature, one can deduce the value of Boltzmann’s constant.
Distinct deviations
The researchers used a gas of ultra-low-density caesium atoms in a vacuum chamber and probed it with a cavity-stabilized microwave laser. They measured the transmission through the cell and recorded the two distinct dips at the positions of the absorption lines. Textbooks have previously modelled the line width as a simple combination of Gaussian and Lorentz distributions, but the precision of the researchers’ measurements revealed small yet significant deviations from this model. Most noticeable, at the level of hundreds of parts per million, were deviations caused by changes in the population statistics of the caesium gas by the laser. “There’s an analogy with speed traps,” explains team member Tom Stace of the University of Queensland. “We’re measuring the speed of the atoms with a Doppler technique. If you look at the traffic just after a speed trap, the cars are all going a little bit slower than they would otherwise have been.” A further, smaller correction arose from unavoidable reflections inside the laser called etalons.
Having corrected for these effects, the researchers extracted and separated the Gaussian component caused by temperature with unprecedented precision, producing an estimate of Boltzmann’s constant consistent with other measurements that has a precision of six parts per million and an uncertainty of 71 parts per million. They are working to improve this further. They also measured the hyperfine splitting of the state with the lowest uncertainty ever recorded. The largest uncertainty in the measurements after the researchers had performed their corrections was the width of the Lorentzian contribution caused by the state’s finite lifetime. The ability to make this measurement could lead to a more precise method for calculating this lifetime. “We can fit [the lifetime width and the thermal width] and derive their values independently from a single measurement,” says Andre Luiten, from the University of Adelaide, who led the work.
De Podesta is impressed, saying the work is important both to fundamental spectroscopy and because “it’s another measurement of Boltzmann’s constant using completely different physics”. “It doesn’t look like the uncertainty will quite be low enough for the measurement to be a significant contributor to the final value, but it adds to the firmness of the foundations,” he says.
The research is described in Nature Communications.