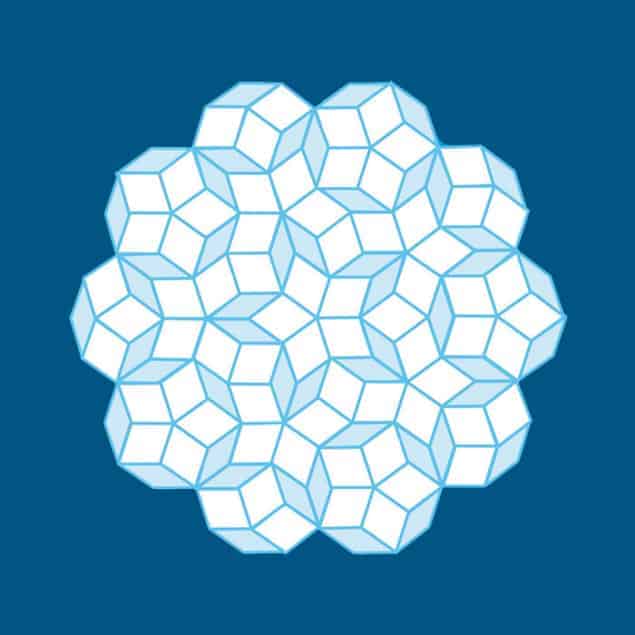
When shrunk to the nanoscale, quasicrystals become plastic. That is the finding of an international team of researchers, which says that its results could potentially widen the material’s applications. Quasicrystals – materials in which the atoms show long-range order but have no finite, periodically repeated unit cell – have fascinated materials scientists ever since their Nobel-prize-winning discovery in 1984. Their practical use, however, has been limited by their brittleness.
Conventional crystals plastically deform through dislocations in their lattice that can allow individual unit cells to swap places relatively easily. This makes some crystals, such as pure metals like copper and gold, highly ductile. In quasicrystals, however, there are no unit cells, so it takes more energy to move dislocations. “Normally, the dislocations in quasicrystals are quite mobile at high temperatures,” says materials-scientist Yu Zou of Massachusetts Institute of Technology in the US. “However, below 500 °C, the dislocations are not that mobile, so this can make the quasicrystal very brittle.”
Doing the “dislocation climb”
The materials often break before they deform, making them hard to form into specific shapes and severely limiting their usefulness. At high temperatures, quasicrystals deform by a process called dislocation climb, in which dislocations move perpendicularly to the actual atomic movement or slip; it remained uncertain, however, whether plastic deformation of quasicrystals was even possible at room temperature without destroying the crystal lattice, and if so what the dislocation mechanism would be.
In 1921 Alan Griffith showed that the fracture strength of materials should increase as samples become smaller, as they can more effectively dissipate strain energy. This phenomenon has since been observed in other materials like ceramics that are brittle at macroscopic scales. Zou, previously at ETH Zurich in Switzerland, and colleagues used this property to increase the stress on a common quasicrystal called i-Al–Pd–Mn until it plastically deformed. A computer simulation suggested that, above sizes of around 500 nm, plastic deformation would preferentially occur by cracking, and the material would therefore quickly fail. At smaller sizes, however, dislocations in the lattice would be able to move without the material cracking.
Catastrophic failure
To test this, the researchers performed two experiments. First, they compressed single-crystalline pillars of i-Al–Pd–Mn ranging from 1.8 μm to 140 nm in diameter until they failed, and observed them using an electron microscope. As predicted, the larger pillars were brittle, with the 1.8 μm pillar failing catastrophically after being compressed by just 3% of its height. However, below 500 nm, the pillars became much more ductile, being compressible by more than 50% without any cracking.
They then tried bending the pillars – the results were qualitatively similar, although the pillars had to be even smaller before they became bendable – a 300 nm pillar snapped rather than bending, for example. This is not surprising, Zou explains, because bending a material stretches one side, and materials are often more brittle in tension than in compression. “If there’s a crack in a sample, compressive stress can make it very difficult for this crack to open and propagate,” he says, “However, if you have a tensile stress, it’s very easy.” Intriguingly, when the researchers examined the micropillars, they appeared to have deformed not by deformation climb but by deformation glide, in which the dislocations move in the same plane as the atomic slip.
“Real breakthrough”
The researchers have subsequently repeated the experiment with another quasicrystal with a different structure and found similar results, so they are confident the results apply to all quasicrystals. Zou suggests the results should lead to studies of quasicrystals at different temperatures. The data also suggest tiny quasicrystals could be useful: they deform elastically at lower stress levels than required to cause plastic deformation, and their high elastic modulus could make them useful for energy storage. In addition, quasicrystals have interesting photonic and electronic properties, so the small-scale plasticity could help engineers to exploit these.
“I think it’s a real breakthrough” says materials-scientist Jean-Marie Dubois of the University of Lorraine in France, who was not involved in the research. “There’s beautiful images of nanorods being deformed by increasing the load – to me, this is the most important part. They also prove that the material remains quasicrystalline during the deformation. The detail of the deformation mechanism is not entirely established, as this requires in situ diffraction studies and things like that rather than post mortem analysis, but at this stage I think it’s quite good.”
The research is published in Nature Communications.