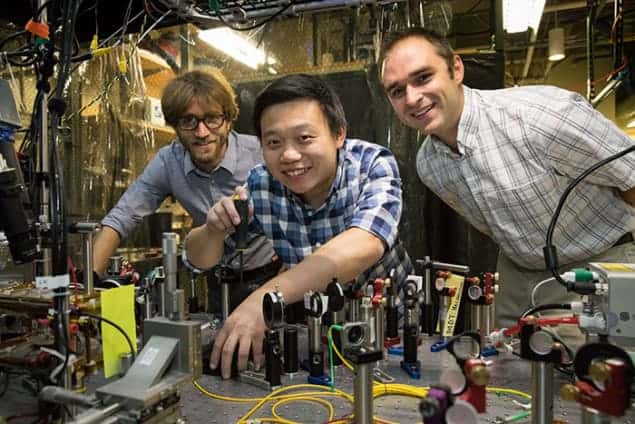
A new way of trapping and cooling atoms has been unveiled by a team of physicists at the University of California, Los Angeles, in the US. The technique uses a pulsed laser known as a frequency comb and could someday be used to study the quantum behaviour of atoms important to biology and astronomy such as hydrogen, carbon, nitrogen and oxygen. Such atoms cannot be cooled using existing methods because that would require high-power ultraviolet lasers, which are not currently available.
Laser cooling was first demonstrated in 1985 and involves slowing the motion of quantum particles such as atoms until their temperature approaches 0 K. Over the past 30 years, the technique has allowed physicists to make precise measurements on ultracold atoms to study quantum processes and even create quantum-logic devices.
In standard laser cooling, multiple lasers are set up such that their beams intersect on a sample of particles such as rubidium atoms. The lasers’ frequencies are tuned slightly below the resonant frequency of the rubidium. Because of the Doppler shift, this results in light absorption primarily by atoms that are moving toward the beam. Each excited atom then emits light in a random direction, resulting in a net loss of momentum, and the process repeats itself, slowing down the atoms.
Chemically interesting
However, current laser technology limits the types of atoms that can be cooled in this way, according to Andrew Jayich, a team member who has since moved to the University of California, Santa Barbara. For example, laser cooling sodium and rubidium is well established, but it has been impossible so far to laser cool the most common atoms found in living things: carbon, oxygen, nitrogen and hydrogen. This is in part because their relevant atomic transitions are in the ultraviolet, and high-power ultraviolet lasers have proven technically challenging to produce. Consequently, scientists still lack a precise understanding of the quantum mechanics of such atoms. The group’s goal is “to extend laser cooling to these chemically interesting species”, Jayich says.
To achieve this goal, Jayich, Wesley Campbell and Xueping Long investigated the cooling potential of a type of laser called a frequency comb. Unlike the continuous-wave lasers used in standard laser cooling that emit a continuous beam at a well-defined frequency, a frequency comb emits short pulses of light over a broad frequency spectrum. This broad spectrum arises from Heisenberg’s uncertainty principle, which specifies that a short pulse must have a large uncertainty in energy, which translates to a large spread in frequency. The frequency-comb spectrum consists of thousands of evenly spaced discrete peaks that look like the teeth of a comb, hence its name.
The trio used their frequency comb to cool a sample of about 10 million rubidium atoms to about 60 μK, which is comparable to conventional laser-cooling methods. Although rubidium is easily cooled using conventional methods, the team has developed a theoretical model that shows that the frequency comb can also be used to cool atomic hydrogen, antihydrogen, carbon, oxygen and nitrogen. Writing in a commentary on the paper in Physics, John Barry of the MIT Lincoln Laboratory points out that laser cooling of hydrogen and antihydrogen could reveal new insights about star formation.
Intermediate state
Instead of using a single ultraviolet photon to excite an oxygen atom, the frequency comb provides two photons of different frequencies whose energies add up to that of an ultraviolet photon. The first photon, from one comb tooth, excites the atom into an intermediate state. Then the second photon, from another comb tooth, excites it from the intermediate state to its final excited state. The many pairs of teeth that are available in a frequency comb ensure that the laser can provide the necessary energy combinations to make the two-step transitions.
Eric Hudson of UCLA – who was not involved in the research – told physicsworld.com: “To me, the most exciting thing about this work is that it is sort of a paradigm shift in thinking.” He added that the new cooling technique will allow the powerful techniques of atomic, molecular and optical physics to be used to solve important problems in chemistry and biology.
The research is described in Physical Review X.
- The basics of how a frequency comb works are explained in this video of Paul Williams of the National Institute of Standards and Technology: “What is a frequency comb?”.