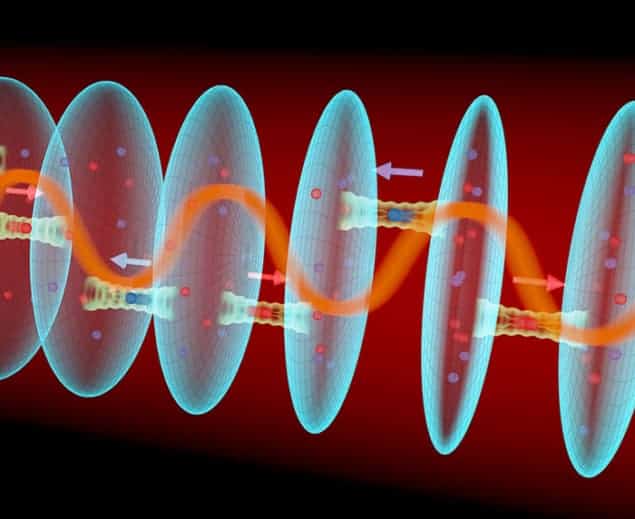
An optical atomic clock has been used by physicists in the US to study the effects of spin–orbit coupling. Spin–orbit coupling is fundamental to understanding how electrons behave within condensed-matter systems and could be exploited in the design of new materials, such as topological insulators and superconductors.
The researchers also plan to adapt their atomic-clock design to study other fundamental phenomena in condensed-matter systems. The work is another example of how physicists have mimicked the behaviour of electrons in solids by fine-tuning interactions between ultracold atoms.
Spin–orbit coupling links the motion of a particle to its quantum spin. For example, an electron has two possible spin states: up and down. Spin–orbit coupling means that otherwise identical electrons with different spins will follow different trajectories in the same electromagnetic field. The consequences of spin–orbit coupling are particularly important in solid materials, where electrons move under the influence of an electromagnetic field produced by a crystal lattice. However, studying spin–orbit coupling directly in materials is difficult because researchers cannot precisely tune a material’s inherent crystal structure during an experiment.
Great potential
Now, Jun Ye and colleagues at JILA, NIST, and the University of Colorado, Boulder, have simulated the behaviour of electrons in a material by using the innards of an atomic clock. Their clock is made of thousands of strontium atoms that have been laser-cooled to nearly absolute zero and trapped in an optical lattice formed from overlapping laser beams. These beams create a grid of potential wells in which the strontium atoms are trapped. The atoms are then bathed in visible light corresponding to an atomic transition in strontium. These excited strontium atoms emit visible radiation, and it is the frequency of this radiation that forms the basis of an extremely precise atomic clock. Indeed, the group has developed strontium clocks that are accurate to within one second for more than 15 billion years.
When the potentials are shallow, the atoms can tunnel
Jun Ye, JILA
But instead of using the clock for timekeeping, the researchers engineered the potential wells to allow the strontium atoms to move around in the optical lattice. “When the potentials are shallow, the atoms can tunnel,” Ye says. The clock atoms offer an additional advantage: they remain in their excited states for a relatively long time, allowing the researchers to observe them for longer.
The team observed that strontium atoms excited to a higher energy level moved differently than unexcited ones. The motion of excited and unexcited atoms in the lattice is analogous to how spin-up and spin-down electrons move through a crystal structure. “Mathematically speaking, the tools used to describe two [atomic] energy levels are completely equivalent to the tools for describing two spin states,” Ye explains.
Mathematically equivalent
This means that while the physicists did not observe actual spin–orbit coupling, they were able to study a mathematically equivalent phenomenon in which the energy state of the atom affects its motion in the same way that an electron’s spin would. Ultracold-atom experiments are a useful proxy for condensed-matter experiments because the atoms can be precisely controlled with lasers in ways that electrons in a material cannot, Ye says.
Their results are “absolutely impressive”, says Tilman Esslinger, a physicist at ETH Zürich who was not involved with the work. Ye and his colleagues’ work offers a first glimpse into a new class of experiments that combine the precision of optical-clock technology with optical lattices, Esslinger adds.
The team’s clock arranges the atoms in a 1D optical lattice. In future work, the researchers are developing a 3D lattice clock that they could use to study spin–orbit coupling and other quantum phenomena in multiple dimensions. This work could be used to develop electronics that rely on quantum spins, or “spintronics,” Ye says.
The research is described in Nature.