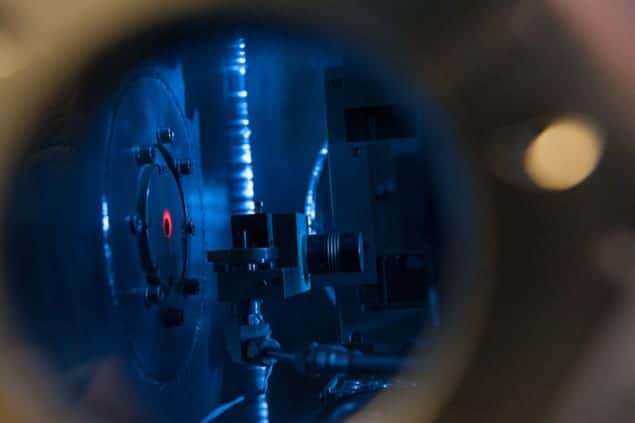
Space radiation has been reproduced in a lab on Earth. Scientists have used a laser-plasma accelerator to replicate the high-energy particle radiation that surrounds our planet. The research could help study the effects of space exploration on humans and lead to more resilient satellite and rocket equipment.
The radiation in space is a major obstacle for our ambitions to explore the solar system. Highly energetic ionizing particles from the Sun and deep space are extremely dangerous for human health because they can pass right through the skin and deposit energy, irreversibly damaging cells and DNA. On top of that, the radiation can also wreak havoc on satellites and equipment.
While the most obvious way to study these effects is to take experiments into space, this is very expensive and impractical. Yet doing the reverse – producing space-like radiation on Earth – is surprisingly difficult. Scientists have tried using conventional cyclotrons and linear particle accelerators. However, these can only produce monoenergetic particles that do not accurately represent the broad range of particle energies found in space radiation.
Now, researchers led by Bernhard Hidding from the University of Strathclyde in the UK have found a solution. The team used laser-plasma accelerators at the University of Dusseldorf and the Rutherford Appleton Laboratory to produce broadband electrons and protons typical of those found in the van-Allen belts – zones of particle radiation caused by Earth’s protective magnetic fields.
After all, radiation in space is one of the key showstoppers for human spaceflight
Bernhard Hidding, University of Strathclyde
Laser to plasma
The accelerator works by firing a high-energy, high-intensity laser at a tiny spot just a few μm2 on a thin-metal-foil target. “The sheer intensity of the laser pulse means that the electric fields involved are orders of magnitude larger than the inneratomic Coulomb forces,” explains Hidding, “The metal-foil target is therefore instantly converted into a plasma.” The plasma particles – electrons and protons – are accelerated by the intense electromagnetic fields of the laser and the collective fields of the other plasma particles. The extent at which this happens depends on the particle’s initial position, resulting in the huge range of energies.
The team studied its plasma particles using electron-sensitive image plates, radiochromic films for protons and scintillating phosphor screens. Then, to prove the lab-made radiation was comparable to space radiation, the team used simulations from NASA. “The NASA codes are based on models as well as a few measurements, so they represent the best knowledge we have,” says Hidding.
Monitoring the damage
The next task was to prove that the system could be used to test the effects of space radiation by subjecting optocouplers to the particle radiation. Optocouplers are common devices that transfer electric signals between isolated circuits. As they are characterized by their current transfer ratio, Hidding and team were able to monitor the radiation-induced degradation by measuring this performance.
The proof-of-concept experiment, described in Scientific Reports, could represent a major breakthrough towards understanding the effects of space radiation without the need to leave Earth. The next step will be to develop a testing standard that can be used to test electronics and biological samples – “After all, radiation in space is one of the key showstoppers for human spaceflight,” Hidding remarks.
Strathclyde’s newly installed laser will also play a key role in future research – “[It is] the highest-average-power laser system in the world today,” says Hiddings. Housed in three radiation-shielded bunkers at the Scottish Centre for the Application of Plasma-based Accelerators (SCAPA), the system will power up to seven beamlines. “The vision is to develop a dedicated beamline for space-radiation reproduction and testing, and to put this to use for the growing space industry in the UK and beyond.”