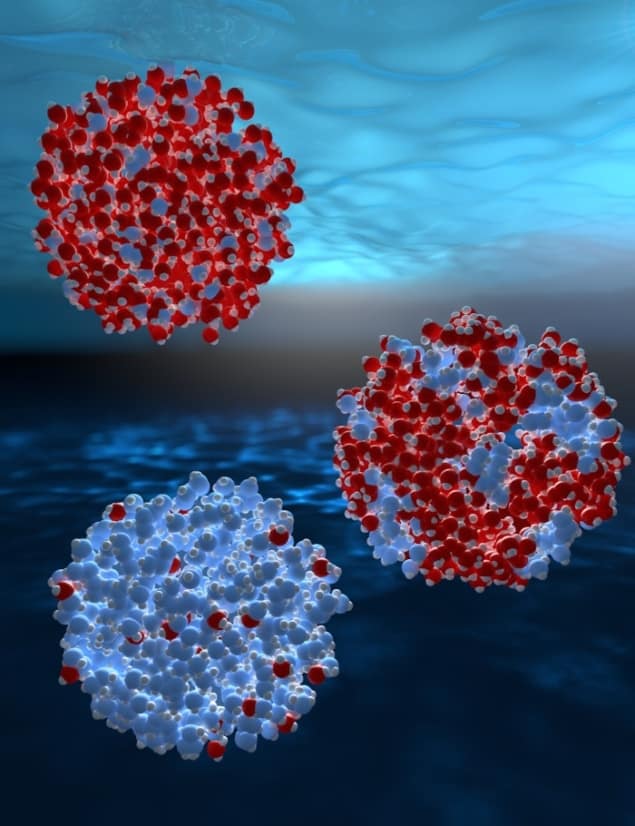
Water could exist in two different liquid phases with different densities. That is the conclusion of researchers in Sweden, Japan and Korea, who have used ultrafast X-ray scattering to measure the properties of supercooled water droplets.
Despite being the most ubiquitous and important liquid on Earth, water is a deeply puzzling substance with physical properties that deviate significantly from those of an idealized liquid. Several theories have been advanced to account for some of water’s idiosyncrasies, but experimental data have been lacking.
Solid ice is the most stable phase of water below 0° C, but the liquid phase remains metastable at sub-zero temperatures. Under normal circumstances, impurities such as dust particles provide nuclei around which ice crystals can form, so freezing occurs quickly. In the laboratory, however, it is relatively easy to supercool liquid water to well below 0° C by removing impurities. As the temperature goes down further, however, molecular motion slows and, below around -40° C, water molecules begin to form crystals around one another, allowing even pure water to crystallize very rapidly.
“No man’s land”
Many theories about liquid water predict a phase transition between a high-density liquid and a low-density liquid at low temperatures. However, this is expected to occur at temperatures and pressures deep inside the so-called “no man’s land” of the water phase diagram where experiments are very difficult to do.
In the new research, Anders Nilsson at Stockholm University and colleagues evaporatively cooled micrometre-scale water droplets by dispensing them into a vacuum. The droplets are then struck by femtosecond X-ray laser pulses, which determine the molecular structure of the water. The temperature that each droplet has reached when it is analyzed is determined by the distance that the droplet travels in the vacuum before it is struck by the X-ray pulse.
Ice crystals formed in many of the droplets, but the researchers identified these from their X-ray diffraction patterns and excluded such droplets from the analysis. By studying the diffraction patterns of the pure liquid droplets, the researchers measured how their compressibility varied with temperature, finding a maximum at around -44° C. A fluid becomes squishier when it is in fluctuating equilibrium between high-density and low-density phases. This is because an increase in pressure can be accommodated by some of the substance transforming from the low-density phase to the high-density phase. The researchers believe this compressibility maximum occurs around the crossover point, where liquid water comprises roughly equal proportions of high and low-density local structures.
At low pressures, this phase transition is not thought to be sharp. Instead, there should be tiny fluctuating regions of one phase within a much larger region of the other phase. If this model is correct, it could explain water’s anomalous properties under ambient conditions because, although water would mainly be in the high-density phase, there would be tiny, fluctuating bubbles of low-density liquid constantly moving around inside it.
Milk, oil and water
By fitting their experimental data to a theoretical model using molecular dynamics simulations, the researchers calculated that, at higher pressures, the crossover point between the two liquid phases would reduce in temperature. The length scale of the density fluctuations would also increase, reaching a “critical point” at a pressure of about 800 atm. “There the liquid would look like milk, because the fluctuations would have reached such a length scale that they would scatter visible light,” says Nilsson. At still higher pressures and lower temperatures, the researchers predict, the high and low-density phases would separate completely so that, at a particular, pressure-dependent temperature, “you would have two different liquids in a glass of water separated with a phase boundary – just like oil and water.”
Paola Gallo of the University of Roma Tre in Italy is impressed with the result. “This group succeeded in going beyond the limit of supercooling obtained before,” she says. “That means that in future we can go even further. There are fields in which it’s important to avoid crystallization: one of these, for example, is cryopreservation. In these cases, for example, solution can be of help, so knowing what is the exact density of water and what is the exact structure water assumes in the supercooled phase is very important.”
Alan Soper of the UK’s Rutherford Appleton Laboratory is intrigued but more sceptical. He notes that the inherent difficulty of measuring the temperature of the droplets makes drawing detailed conclusions difficult, and says the increase in compressibility the researchers record is tiny: “It’s just a very small increase in the scattering and it goes through some sort of maximum… It’s just as likely that it’s just going into the phase where it’s about to crystallize and the molecules are rearranging so they can form the crystal,” he says. “They’ve clearly seen something and it’s very interesting,” he concludes. “But what’s actually causing it is probably something we don’t have a straightforward answer to.”
The research is described in Science.