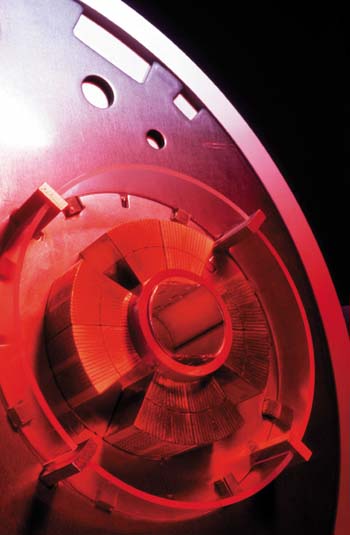
The phrase “needle in a haystack” – the challenge of finding something small but important in the midst of a much, much larger object – is often used to describe CERN. It could be applied to searching for someone’s office in one of the 373 buildings that occupy the laboratory’s site at Meyrin, just outside Geneva on the Swiss-French border, but the real needle in the haystack at CERN is the Higgs boson.
The Higgs is the particle that is responsible for other fundamental particles such as quarks and Z-bosons having mass. It is also the main reason that CERN is building a machine called the Large Hadron Collider (LHC) at a cost of SwFr 2bn. Particles predicted by supersymmetry – the theory that every particle has a supersymmetric partner with the same mass and charge but different “spin” – are the other top priority.
There is a chance, albeit it a small one, that particle physicists might find the Higgs boson at CERN’s large electron-positron (LEP) collider before it is shut down to make way for the LHC. It all depends on how high the beam energy at LEP – which currently stands at 103 GeV (103 X 109 electron volts) – can be raised. “1 GeV can matter at this stage,” says Luciano Maiani, the lab’s director general. “Exploring up to a mass of 114 GeV is optimistic but not impossible. Unless we see the Higgs, the current plan is for LEP to be dismantled after it stops running at the end of September. Installation of the LHC in the LEP tunnel will then start in October.”
Big science
The LHC will collide protons at a centre-of-mass energy of 14 TeV (14 000 GeV), and two massive general-purpose detectors – ATLAS and CMS – will search for evidence of the Higgs and supersymmetry. A third experiment called LHC-b will probe the difference between matter and antimatter, while the ALICE experiment will study the extreme state of matter known as the quark-gluon plasma.
So will the LHC start in 2005 as planned? “Both the schedule and the budget seem to be under control,” says Maiani, “although there are some delays in the civil engineering for the ATLAS and CMS caverns. We will assess any impact on schedule at the end of this year.”
Industrial production is now the big unknown according to Maiani. Industry has to make 1200 dipole magnets, each 15 metres long, and they have to be installed in a 27 km circumference on a tight schedule. “This is a gigantic job but we must succeed,” he says. “With the LHC we are putting our credibility as a community on the line. I am confident that the CERN staff will cope with this difficult task.”
Whether the staff agree is a different matter. A poster for a recent staff meeting about “employment conditions and staff reduction” at CERN showed a pole vaulter trying to jump over a bar labelled LHC, but the director general was sawing off the bottom of the pole. How did Maiani react to that? “We are in the middle of a five-yearly review of employment conditions and this may explain some agitation,” he explains, reluctant to comment any further.
Detector developments
Meanwhile, two massive teams of physicists are preparing the two detectors for the LHC. Both ATLAS and CMS have the same basic structure: an inner tracker to measure the paths of electrically charged particles; a calorimeter to measure the energy of charged and neutral particles; and a spectrometer to track muons, the only particles apart from neutrinos that will reach the outer region of the detector. Both collaborations involve more than 1700 physicists from more than 30 countries.
The 12 500 ton CMS detector is budgeted to cost SwFr 452m at 1995 prices, and will have the biggest superconducting solenoid magnet ever made. The construction of the magnet will drive the rest of the schedule, says CMS spokesperson Michel Della Negra. The magnet will be tested around March 2004 and, if all goes well, the detector will be lowered 100 metres below ground.
A major challenge for CMS is its scintillator crystals. The L3 detector at LEP used bismuth germanium oxide (BGO) crystals, explains Della Negra, but BGO is too slow for the LHC and would not survive the intense radiation levels either. That is why a new crystal material, lead tungstate, had to be developed specially for the LHC. Moreover, CMS needs 11 m3 of lead tungstate – about 80 000 crystals – whereas L3 needed only 1 m3 of BGO. Half of the crystals will come from Russia and half from China. However, changes in the exchange rate between the dollar and the Swiss franc mean that the current cost of crystals in Swiss francs has risen by 30% – just one of a variety of problems that the project leaders have had to face.
One of the major challenges when building the detectors is to ensure that the electronics for the inner tracker can survive the intense radiation produced there, and also take up as little volume inside the detector as possible, explains Peter Jenni, spokesperson for ATLAS. Many of the components for ATLAS have already been built, he says. For instance, 12 of the 64 modules needed for the barrel of the hadronic tile calorimeter have been completed and are now at CERN. ATLAS is budgeted to cost SwFr 475m.
Both collaborations are also now looking closely at the issue of computing and how to cope with the enormous amounts of data that will flow from the LHC detectors. Indeed, this challenge is the driving force behind the development of a new approach to computing called the Grid.
We have the technology
Although fundamental physics is CERN’s top priority, the lab has started to pay more attention to technology transfer in recent years. “Technology transfer has always happened at CERN, but now it is more proactive and explicit,” says Juan Antonio Rubio, head of CERN’s newly created education and technology transfer division. “The aim is less to generate revenue,” he says, “than to demonstrate to the CERN member states that the money invested in CERN is bringing benefits to European industry.”
Technologies that CERN is strong in include superconductivity, information technology, control systems and vacuum technology. Various accelerator and detector technologies have also been transferred to medical applications. For example, the BGO crystals developed for the LEP detectors are now being used in PET scanners.
But the most important area of technology transfer is people, says Rubio. This currently happens at many levels, from placement students who spend a summer at the lab to fellows who have spent several years at CERN. Another area is collaboration agreements with companies, says Rubio, and CERN is currently undertaking a study to find out what mechanisms work best for such collaborations.
The future
It has been suggested that CERN will find it difficult to maintain the intellectual life of the lab during the gap between LEP and LHC. Maiani disagrees. “Analysis of LEP data will take two or so years,” he says, “leaving only a three or four year gap before physics results start to pour in from the LHC.” He also points to on-going experiments with protons, muons, neutrinos, neutrons, kaons and the antiproton decelerator.
So what will happen at CERN after the LHC? Maiani sees three possibilities. The first is a linear collider called CLIC that will have a centre-of-mass energy of 3 or 4 TeV. Maiani does not see CLIC as being in competition with existing proposals to build a 1 TeV linear collider in Germany, Japan or the US. Such a collider would, he says, come on-line before CLIC and focus on a lower energy range. “However, money is money,” he admits, and if it came to a choice between a 1 TeV machine and a 3-4 TeV machine, he would back the latter.
The second option is a neutrino factory based on a muon storage ring. Maiani thinks that any neutrino factory would be built by a network of labs, and with the detectors thousands of kilometres away from the storage ring, location will not be too important. “It will be really a world machine,” he says.
The third option is a second phase of the LHC. Maiani believes that as microelectronics moves to shorter length scales, it may be possible to cope with even higher collision luminosities, thereby increasing the accessible energy range. Better magnets could also allow the energy to be increased beyond 14 TeV.
However, Maiani recognizes the dangers of particle physics becoming isolated from the rest of science and society, and sees it as one of his jobs to make sure that this does not happen. “The particle-physics community needs to be more open,” he cautions.