Shahram Vaezy, Roy Martin and Lawrence Crum received the 2002 Science Writing Award for Professionals in Acoustics from the Acoustical Society of America for this article.

Four years ago the world was stunned to learn that Princess Diana had been killed in a high-speed car crash in Paris. The princess died from internal bleeding that stemmed from major chest, lung and head injuries. Her life might have been saved, however, had paramedics been able to treat her hidden wounds at the scene of the accident. Indeed, internal bleeding is the real killer in many accidents. That is why our group at the University of Washington in Seattle is developing a portable device that is designed to detect and stop internal bleeding using ultrasound.
Doctors and medical practitioners have known for centuries that bleeding can be stopped by applying intense heat. Hot irons, for example, were once used to cauterize wounds sustained on the battlefield. In modern operating theatres, however, surgeons stop injured blood vessels from haemorrhaging by passing an intense electric current through the tissue – a technique known as electrocautery. In both cases, the changes in the structure of the blood vessels and the surrounding tissue are responsible for stopping the haemorrhage. When heat is applied, the blood vessels first shrink, then blood clots form and, finally, the vessels shut off so that blood cannot flow through them.
A sound advantage
Given the number of different methods available to stop bleeding, why do we need another? What advantages does ultrasound offer? All the techniques that are in current clinical use can only stop the bleeding from wounds close to surface of the skin. Lasers and electric currents, for example, can only penetrate some 2–3 mm of tissue. Meanwhile, tourniquets and clamps constrict the flow of blood through the organ or limb as a whole, and run the risk of starving both the healthy and wounded tissue of blood and oxygen, thus causing further damage. None of these techniques are capable of stopping internal bleeding.
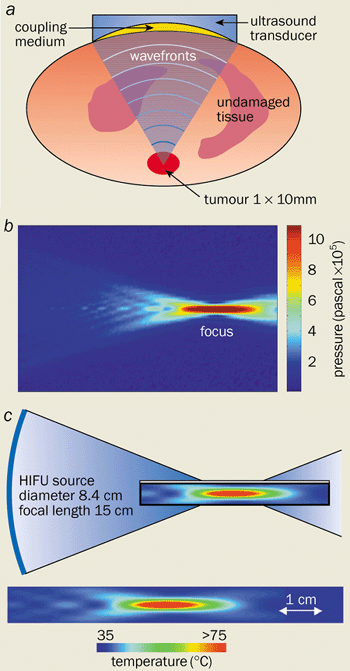
Ultrasound, on the other hand, can penetrate soft tissue easily and can deliver sufficient energy to cauterize blood vessels located deep within the body (figure 1a). The acoustic energy can also be sufficiently focused so that the injured region can be treated while leaving the healthy tissue unharmed. No other technique can rival the ability of ultrasound to penetrate tissue and to be focused tightly. This technique, known as acoustic haemostasis, thus offers a non-invasive treatment for internal bleeding.
By focusing high-power ultrasound, large amounts of energy can be delivered to specific sites deep within the body. Indeed, the intensity at the focal point is typically 1000–10,000 W cm-2, some four to five orders of magnitude greater than the intensities of diagnostic ultrasound. The waves can be focused using a concave transducer that emits ultrasound waves that converge to a region the size and shape of a grain of rice. The pressure that is generated by the acoustic waves is as high as 1 million pascals at the focal point, but much lower in the surrounding tissue (figure 1b). Such high pressures lead to an intense acoustic beam that produces high temperatures at the focal point due to the absorption of the waves and the conversion of mechanical energy to thermal energy (figure 1c).
How to stop bleeding
So far, investigations into the use of acoustic waves to halt bleeding have concentrated on two very different frequency ranges. Surgeons can currently control bleeding in the operating theatre using a device called a harmonic scalpel, a metal applicator that produces low-frequency ultrasound at 55 kHz. The device, which has been developed by the surgical-instrument manufacturer Ethicon Endo-Surgery Inc., exploits the frictional force between the vibrating tip and the surrounding tissue. The heat that is generated can stop bleeding within a minute, but only at the areas in direct contact with the tip. As such, harmonic scalpels can only treat surface bleeding. Nevertheless, they have found a variety of applications in liver surgery, hysterectomy operations and other surgical procedures.
Our group, however, is concentrating on high-frequency acoustic waves in the 1-10 MHz range. The main application we envisage is in the treatment of bleeding from injured organs and blood vessels. Our studies have concentrated on the liver and spleen, the two organs that are damaged most frequently in trauma. Both organs contain large numbers of blood vessels, which makes it particularly difficult to control bleeding.
Simple wounds to the liver can be treated with electrocautery and biochemical sealants. However, bleeding from more complex injuries is usually stopped by plugging the wound with absorbent material. One disadvantage of this treatment is that the patient needs a second operation some 24-72 hours later to remove the absorber. Meanwhile, the only way to treat patients whose spleen has been severely damaged is to remove part, or even all, of the organ. Surgeons, however, are beginning to reconsider such drastic action because of the important role that the spleen plays in removing worn-out blood cells and other waste material from the blood stream, and because of the risk of post-operative infection. High-intensity focused ultrasound has been demonstrated to effectively stop bleeding from wounds to the liver and spleen of animals, and has the potential to treat humans as well.
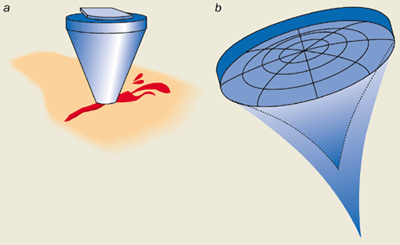
Our group has used ultrasound transducers at various frequencies (2-5 MHz) and at intensities between 1000-3000 W cm-2 for haemostasis. The transducers are concave piezoelectric crystals that convert an oscillating electric field into a mechanical vibration, which leads to a converging acoustic field. These devices were originally housed in cone-shaped applicators that were filled with water so that the acoustic energy could couple effectively to the tissue (figure 2a).
At first we scanned the focus across the injured surface by manually moving the ultrasound transducer, and brought the bleeding from an incision in the liver under control within 90 s. No bleeding occurred within three hours after the treatment, and we believe that the wound would have stayed “dry” for much longer had we prolonged our experiments. Indeed, recent longer-term studies have demonstrated that no re-bleeding occurs and that the wounds heal as normal.
High-intensity focused ultrasound has also been used to treat bleeding incisions and complex injuries in the livers of pigs. The bleeding from cuts some 50 mm in length and up to 8 mm deep can be brought under control in less than 30 s, and it can be stopped completely within one minute.
Bleeding from major arteries and veins that are several millimetres in diameter has also been investigated. By focusing continuous high-frequency acoustic waves, our group has found that the bleeding from major blood vessels that had been punctured with a thick needle can be stopped in about a minute. We have also looked at more severe cases in which blood vessels had been slashed longitudinally. Such extensive injuries meant that the acoustic energy had to be spread over the entire length of the laceration, some 2-8 mm. We therefore developed a mechanical scanning system to oscillate the point at which the acoustic waves were focused some 15-25 times per second. On average, it took 25 s to completely stop the bleeding.
Interestingly, the treatment time did not change significantly when the animals were injected with a short-acting “anti-coagulant” agent, a drug that thins the blood and thus prevents clotting. This result suggests that acoustic waves can inhibit bleeding independently of the series of biological reactions involving the proteins and enzymes that lead to the formation of blood clots. Alternatively, high-intensity focused ultrasound may accelerate these reactions even when the anti-coagulant is present.
Physical mechanisms behind acoustic surgery
In 1997 our group found that different biological mechanisms stop bleeding in different types of tissue exposed to ultrasound. For example, small blood vessels in the liver collapse, while lacerations in much larger blood vessels fuse to stop the bleeding. These effects may be associated with specific thermal and mechanical mechanisms.
A large number of the structural changes observed in tissue that has been treated with high-intensity focused ultrasound are directly related to the intense heat that is produced when the energy is absorbed. Indeed, the temperature of liver tissue rises to as high as 80 °C at the focal point. As a result, water escapes from the tissue causing the organs to shrink and the blood vessels to collapse under the structural stress and strain. Thermal effects might be responsible for the closure of small blood vessels up to 2 mm in diameter, but they also appear to stem bleeding from major veins. In this case, the surface walls of these blood vessels appear to thicken and an insoluble protein called fibrin precipitates from the blood to form a network of fibres that promotes clotting and acts as a sealant for the wound.
Many of these structural changes are also observed in other techniques that rely on thermal effects, such as laser treatment and electrocautery. However, acoustic therapy also appears to induce a range of mechanical mechanisms that prevent bleeding, including “acoustic streaming” and “cavitation”.
When an intense sound wave passes through a bulk fluid, the absorption and scattering of the energy by the liquid leads to strong and constant flow. Indeed, if an ultrasound wave is directed towards the surface of a fluid, this so-called streaming mechanism produces a fountain. Acoustic streaming brings practical benefits when it comes to stopping bleeding. When the focus of the high-intensity ultrasound is positioned over a bleeding wound, streaming pushes the blood away from the injury, either back into the blood vessel or to the sides.
This action has two advantages. First, it gives surgeons a far better view of the wound since there is no pool of blood to obstruct it. Second, it means that more thermal energy can be applied to the injury itself, thereby accelerating the haemostasis process. The absence of blood surrounding the wound also makes it easier for the walls of the blood vessels to fuse together.
The second mechanical mechanism that appears to stop bleeding is cavitation. The rapid pressure changes induced by ultrasound lead to the formation of cavities or bubbles in the blood. Cavitation was initially thought to have the same damaging effect on blood vessels as it does on the propellers of ships. When the intensity of the ultrasound increases above a certain threshold, the sudden growth and collapse of the bubbles causes extreme pressure changes that can drill holes in the tissue or blood vessels. However, Kullervo Hynynen and co-workers at the Massachusetts Institute of Technology and Harvard University, plus our group, have observed an important new role for cavitation. When the intensity, frequency and duration of the ultrasound pulses are tuned to specific values, the waves can break down the tissue structure to produce tissue “homogenates” that tend to clump together and act as a sealant.
Spreading these tissue homogenates over the injury also seems to seal the wound. Indeed, our group has found that this seal is particularly effective at healing injuries to the spleen that could not be treated using high-intensity focused ultrasound to close the blood vessels. The seal initially looked like a paste covering the wound, but it soon turned into a hard scab that eventually healed as normal.
Many clinicians were worried that the high levels of heat produced by focusing high-intensity ultrasound within tissue would have adverse effects on the blood itself. Recent studies by Sandra Poliachik of the University of Washington at Seattle and co-workers have shown that bursts of ultrasound lasting up to 1 s and with intensities as high as 2000 W cm-2 produce a negligible temperature rise in the blood. Moreover, no changes in the number of blood cells, white blood cells or platelets were observed. However, this situation changed dramatically when a so-called contrast agent was added to the blood. Bubbles in the blood appeared and some 45% of the blood cells were destroyed.
Contrast agents are chemical substances that are used to make ruptured organs easier to identify. These results show that a great deal of care is needed when treating a patient who has been given a contrast agent with high-intensity focused ultrasound, otherwise the sound waves could cause further clinical damage.
Surgical instruments
When it comes to treating bleeding wounds with sound waves, there are two important practical aspects to consider. Clinicians need to ensure that the ultrasound energy couples well to the tissue and that the high-intensity beam can be precisely focused onto the injury in a controlled way.
To satisfy the first of these criteria, the sound waves have to travel from an ultrasound transducer to the focal point through a homogenous medium that has low attenuation and an acoustic impedance that almost matches the tissue under treatment, otherwise the ultrasound is reflected back towards the transducer. Traditionally, water has been the material of choice because it has all the desired acoustic characteristics. Indeed, the majority of acoustic-haemostasis studies have been carried out using a high-frequency ultrasound transducer that is housed in a water-filled plastic cone with a polyurethane membrane at the tip.
In spite of the successful results, there are several drawbacks with water-filled devices. Extra equipment is needed to de-gas and circulate the water to prevent the formation of air bubbles that would scatter the sound wave and spoil the transmission of the acoustic energy. Moreover, the membrane at the tip can easily be damaged, the equipment is cumbersome to use in remote locations, and it is difficult to ensure that it remains sterile.
Our group is investigating different designs of couplers and other materials that could overcome the shortcomings of water coupling. New applicators have been developed that use piezoelectric elements bonded onto aluminium cones that guide and couple the ultrasound energy to the tissue. The cones can have various shaped tips that produce different ultrasound patterns, depending on the type of injury that is being treated. Another approach uses variously shaped devices made from polymer-based gels, which are disposable. One advantage of these couplers is that the concentration of the polymer can be varied so that it matches the acoustic impedance of the injured tissue, thus providing a highly efficient method for transferring the acoustic energy to the tissue. Preliminary results show that the polymer and metallic couplers are at least as effective as water-filled devices at stopping bleeding from injuries to the liver, spleen and arteries.
The other crucial factor is the ability to position the focal point of the high-intensity ultrasound waves precisely. This is important because clinicians need to be able to direct the energy at the bleeding wound to optimize the treatment and to prevent damage to the surrounding tissue. But such dynamic focusing has proved both challenging and time consuming with an ultrasound transducer that consists of a single element with a fixed focus. Hynynen at MIT and Emad Ebbini at the University of Minnesota, among others, have shown that the problems can be overcome with devices that have several transducers and a variable focus (figure 2b). By adjusting the phase of the electrical signal to each of the transducers, clinicians can vary the position of the focal point dynamically without physically moving the ultrasound transducer.
Guiding and monitoring acoustic surgery
Not surprisingly, acoustic surgery can be performed more quickly and effectively when the ultrasound beam is focused accurately on the wound. Bleeding from punctured blood vessels, for example, can be stopped within 3 s if the energy can be focused precisely.
Clearly it helps to monitor the position of beam within the body. In 1999 our group developed a so-called Doppler-guided system to target bleeding vessels. Ultrasound waves reflected from blood gushing out of a ruptured vessel are Doppler shifted to lower frequencies. In contrast, no frequency shift occurs if the beam is reflected from an intact artery. Our system produces an audible sound when the focal point of the high-intensity focused ultrasound is placed directly over a bleeding wound. Indeed, no sound is heard if the focus and the injured site are misaligned by as little as 1 mm. Using Doppler guidance, we found that we could control and completely stop the bleeding some five times faster than by aligning the transducer and wound by eye. Moreover, the technique might also provide a valuable method for monitoring treatment, as the distinct Doppler sound disappears as soon as the bleeding has stopped.
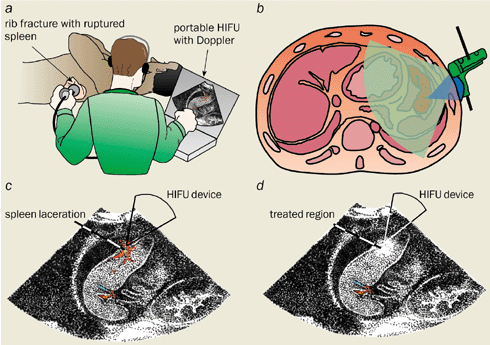
Diagnostic ultrasound is used routinely in hospitals to image internal organs. It also offers a valuable method of targeting bleeding arteries and monitoring them thanks to its real-time-imaging capabilities. Moreover, diagnostic ultrasound is easy to use and can readily be combined with high-intensity focused ultrasound. Our group has devised and tested an image-guided device to treat bleeding by synchronizing bursts of high-intensity sound waves with an ultrasound scanner that produces 2D images of the body. The high-intensity transducer was configured so that the energy was focused in the image plane of the scanner, where it appeared as a bright spot (figure 3).
The technique looks promising, particularly as other ultrasound-imaging and magnetic-resonance-imaging methods do not provide real-time results, which means that patients have to lie perfectly still. Moreover, microscopic examinations have confirmed that no cellular damage occurs in the region where the bright spot appears if the ultrasound has a low intensity. The results suggest that low- and high-intensity sound waves could be used to guide the beam without damaging tissue and for monitoring the treatment.
Image-guided ultrasound has been used to treat injuries to the femoral artery (the main artery in the thigh), wounds left behind when a catheter is removed and blood vessels in the pelvis – all non-invasively.
Clinical and therapeutic applications
The therapeutic applications of high-intensity focused ultrasound were first envisaged by John Lynn in the 1940s, and were later pursued by William Fry, a physicist at the University of Illinois at Urbana Champaign, and his brother Francis, together with Padmakar Lele at MIT. A few years later, the first clinical trials took place in which high-intensity ultrasound was used to treat Parkinson’s disease by creating tiny lesions in parts of the brain that were inaccessible to surgeons. But there is a raft of other possible applications for high-intensity ultrasound.
It could be used to treat internal bleeding in patients who have suffered a major accident in a remote location, on their way to hospital, or even in the emergency room. Indeed, acoustic surgery could save the lives of patients during the “golden hour” – the grace period after a major accident – when steps must be taken to stop casualties from bleeding to death. Ultimately, high-intensity ultrasound might be able to heal wounded organs without the need for invasive surgery.
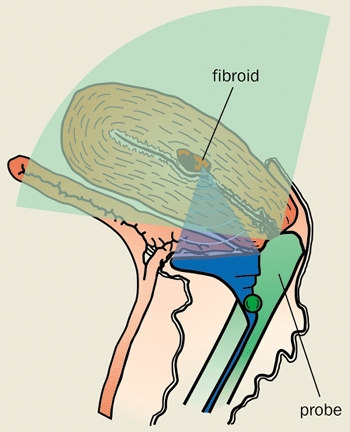
Further possible applications of ultrasound might include cauterizing tissue that is about to be surgically removed in order to reduce the amount of bleeding during an operation. In addition, Gail ter Haar and co-workers at the Royal Marsden Hospital in the UK are aiming to use high-intensity focused ultrasound to treat a syndrome in unborn twins in which there is an imbalance of blood flow between the two babies. Such a condition is often fatal without treatment, but ultrasound could be used to narrow the appropriate blood vessel joining the twins.
It may even become possible to treat tumours by closing the main artery that supplies them with blood using acoustic energy. Other techniques that have been developed to close arteries have demonstrated that the tumours do indeed shrink. However, high-intensity ultrasound appears a more attractive method because it is non-invasive.
Currently, sound waves are used to treat prostate cancer and benign enlarged prostates. Clinical trials are also under way for the treatment of breast tumours and cancers of the kidney, ovaries and liver. Meanwhile our group is developing an image-guided instrument to treat fibroids of the womb without the need for surgery (figure 4).
Studies so far have shown that the spread and re-growth of tumours is significantly reduced after ultrasound treatment and that there are no adverse side effects. Indeed, in a number of studies – including ones by ter Haar at the Royal Marsden – the patients have been treated without an anaesthetic, while the ultrasound transducer was placed against the skin. Although some clinicians are worried that high-intensity ultrasound could cause tumours to spread from one part of the body to another, there is clear evidence to refute this concern.
A sound future
Acoustic therapy has enormous potential to stop bleeding and treat tumours deep within the body in a safe and non-invasive way. The main advantage of acoustic therapy is its ability to treat tissue with sub-millimetre accuracy without damaging the surrounding body. High-intensity ultrasound stands to gain further clinical acceptance and promises to advance the trend towards minimally and non-invasive medical treatment.