The fortuitous alignment of a quasar and a distant galaxy has enabled astronomers to unravel the origin and evolution of chemical elements
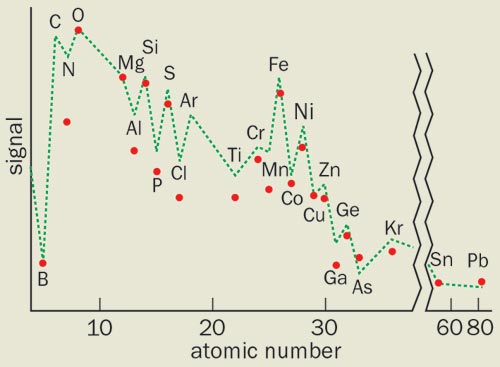
Cosmologists and fossil hunters have more in common than it might first appear. Palaeontologists analyse fossil records of the last 3.5 billion years to track the evolution of life on Earth. Motivated by a similar curiosity, astronomers are searching for chemical elements in stars and cosmic gas to understand the origin of the chemistry of the universe, and how it has developed since the Big Bang.
The basic theoretical framework of the universe’s chemistry has been in place for half a century. The lightest elements – hydrogen and helium, along with trace amounts of deuterium and lithium – were created within a few minutes of the Big Bang. All of the other elements were assembled at much later times by the fusion of hydrogen and helium nuclei in the interior of stars. Some of these elements were dispersed through space in the violent supernova explosions with which some stars end their lives. They were then condensed into subsequent generations of stars, and into the planets that formed around them. Some of these elements were eventually incorporated into organic structures and became essential for life on Earth.
This cosmic cycle continues to the present day. The closest region of active star formation is the Orion nebula, which is visible to anyone who looks up at the sky on a clear winter’s night. But the details of the cycle are still far from clear. For example, we do not know when most of the elements were produced in the universe as a whole, or which stars were responsible for producing which elements. In order to unravel the path of chemical evolution we have to shift our attention to much further away than the Orion nebula.
Lucky alignment
Traditionally, astronomers have looked for clues about stellar chemistry in stars of different ages and at different locations within our galaxy – the Milky Way. Stars in the halo of the Milky Way probably formed a very long time ago. Those that are still around us bear the imprint of the chemical composition of the protogalaxy in their atmospheres, like living fossils of a bygone era in the history of the Earth. But this is only part of the story. The Milky Way is just one of several billion galaxies and its stellar population therefore gives us only a limited window on the process of galactic chemical evolution.
Thanks to recent technological advances, astronomers have now begun to extend their studies of chemical abundances beyond the Milky Way. In a major development, we are now able to see nearly all the way back to the Big Bang. Large telescopes, such as the Very Large Telescope at the European Southern Observatory in Chile and the Keck telescopes on Hawaii, are so efficient at gathering and recording light that astronomers are able to study galaxies at the edge of the observable universe.
The light that falls on these telescopes left some galaxies when the universe was just 1 or 2 billion years old. We know this from its redshift – an increase in the apparent wavelength of the light due to the expansion of the universe. These galaxies therefore appear not as they are today, but as they were 12 billion years ago – just what the galactic fossil hunters are looking for.
Some of the galaxies can be seen directly, while others are only observable by the shadow that they cast in the light of more distant and brighter objects. These two techniques for studying high-redshift galaxies are highly complementary.
When a galaxy is bright enough that its starlight can be seen directly, we can use spectroscopy to discern its chemical composition and are able to relate it to nearby galaxies around us today. However, the furthest galaxies are generally too faint to reveal their chemical composition in detail. For this we need to use high-resolution spectroscopy. But this is only possible when the light from something much brighter – such as a quasar – shines through the galaxy, thanks to a chance alignment of the two objects as seen from Earth.
One such alignment was recently discovered by a team of astronomers in the US. Jason Prochaska of the University of California at Santa Cruz, and Chris Howk and Artie Wolfe at the University of California in San Diego have been systematically searching for such galaxy-quasar pairs and following up the most promising candidates with the Keck telescopes. This is the first pair that they have found in over a 100 candidates (Nature 423 57-59).
The pair consists of a quasar that shines through a dense region in a foreground galaxy, which means that the number of atoms available to absorb the quasar light is unusually large. The spectral signatures of the quasar occur in a different part of the spectrum to those of the foreground galaxy due to their different redshifts. The spectral lines of the quasar are also broader than those of the intervening galaxy because they are produced by a hot, as opposed to the cold galactic, gas. The team found that the intervening galaxy is surprisingly advanced along the path of chemical evolution.
Elemental abundance
The redshift of the galaxy is 2.626 – which corresponds to looking back in time some 12 billion years. Stars in our galaxy that are this old contain only small amounts of chemical elements, typically just one-tenth to one-hundredth of the amounts in the Sun. This is generally taken as a sign that little star formation took place in the proto-Milky Way.
But we now have a quite different example of a galaxy that underwent a significant amount of star formation in just 2.5 billion years, during which its chemical composition became similar to that of the Sun. In particular, the abundance of oxygen in the stars of this galaxy had already grown to one-third of the solar value.
The Milky Way is a spiral galaxy and observations show that in most “spirals”, star formation has proceeded at a relatively steady pace over their entire existence. But there are other galaxies in the nearby universe that astronomers suspect have squandered most of their resources in their youth, by forming stars at much faster rates. These are the elliptical galaxies, which have very little gas left and contain a predominantly old collection of stars.
Prochaska and colleagues may well have found the progenitor of one of today’s elliptical galaxies, and observed it at an early stage of its evolution. The importance of such a link between the past and the present, and between different classes of galaxies, is hard to overestimate.
It tells us that galaxies formed stars at different rates and at different times. Some formed slowly and at an even pace, while others were born in one major episode of star formation that took place a long time ago. This is what we had suspected from the way galaxies look today, but Prochaska and co-workers have found a concrete example that confirms some of these ideas.
Furthermore, the rare combination of high gas density and high element abundances in this particular quasar-galaxy pair produces an extraordinarily rich spectrum. This brings within reach a number of atomic transitions that are normally far too weak to be detected. Prochaska’s team has measured 25 chemical elements in this distant galaxy ranging from the relatively light elements boron and nitrogen to real heavyweights such as tin and lead (see figure).
Extragalactic heat
Many of these elements had never been seen before outside the Milky Way. They therefore provide an unprecedented opportunity to study the complex pattern of element abundances in a galaxy that is in a very different place and time in the universe.
This pattern is surprisingly similar to that in the Sun, which is a strong clue that the same basic physical processes that synthesize chemical elements inside stars here and now were operating there and then. It also means that some universal law – which is roughly invariant in time and space – must determine the relative numbers of stars of different masses that form in a galaxy. Stars that have different masses produce different amounts of each chemical element, yet the final mix seems to be approximately the same. Since stars of different masses synthesize different elements in different proportions, this need not be the case.
The challenge now is to extend this type of measurement to galaxies with even higher redshifts. This will allow astronomers to look for chemical traces that were left over from the very first generation of stars to form in the universe – perhaps only a few 100 million years after the Big Bang.
These redshifts are so high that the wavelength of the starlight will be shifted not into the visible region – as in the galaxy studied by Prochaska and collaborators – but all the way into the infrared. Advanced plans are already in place to construct near-infrared spectrographs for most large telescopes, and the first of these will be in operation in just a couple of years.